Calculating Surface Tension from Contact Angle Explained
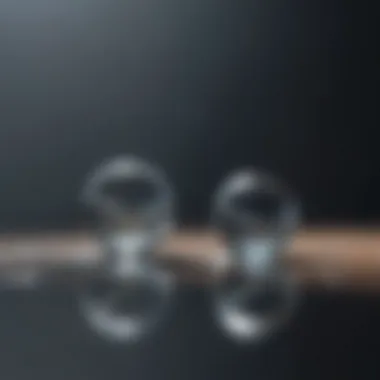
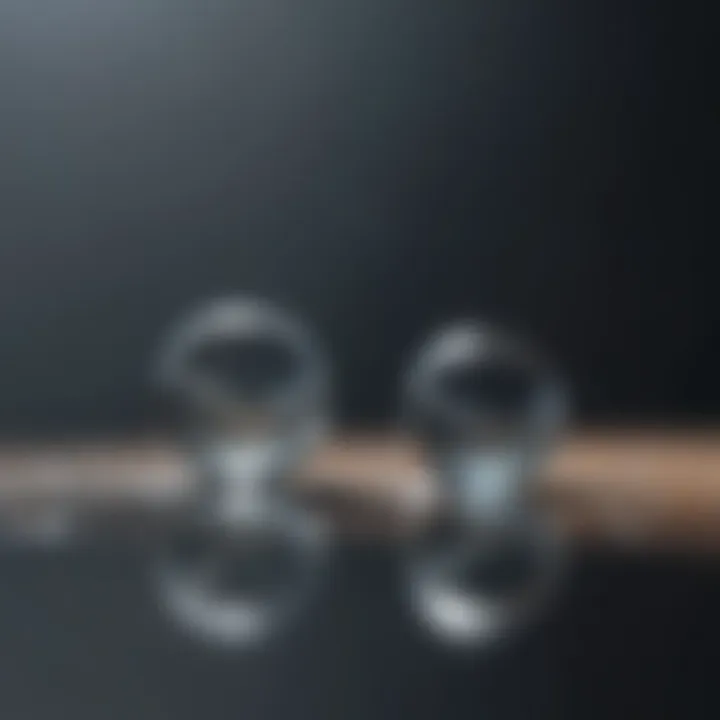
Intro
Understanding the relationship between surface tension and contact angle is crucial for fields like material science, fluid dynamics, and even biological systems. This intricate connection not only influences how liquids interact with solids, but also has significant implications in various applications from coating processes to biological interactions.
The need for a reliable methodology to calculate surface tension based on observed contact angles is apparent. By grasping the principles that govern these phenomena, researchers and industry professionals can better predict and manipulate surface interactions. Whether you're designing a new polymer or improving adhesive properties, knowing how to measure and interpret these factors can make all the difference.
In the sections to follow, we will thoroughly investigate the current state of research, laying out foundational concepts alongside cutting-edge insights. Our aim is to provide a comprehensive resource for anyone looking to deepen their understanding of this vital topic.
Foreword to Surface Tension and Contact Angle
Understanding surface tension and contact angle is not just essential but foundational for many scientific and industrial applications. These concepts are closely linked to how liquids interact with solid surfaces, influencing everything from paint adherence to the way raindrops behave on leaves. In a nutshell, surface tension describes the elastic-like force existing at the surface of a liquid, while the contact angle reflects how a liquid droplet maintains or loses shape when in contact with a solid surface.
Defining Surface Tension
At its core, surface tension can be defined as the tendency of liquid surfaces to shrink into the minimal surface area possible. It arises from the cohesive forces between the molecules within the liquid, which act differently at the surface than for those in the bulk. These forces keep the molecules at the surface more tightly packed, leading to phenomena such as water beads on a waxed car or the ability of small insects, like water striders, to walk on water.
For instance, when water meets oil, the surface tension plays a crucial role. Water's high surface tension results from strong hydrogen bonds amongst its molecules, creating a compelling barrier where the two liquids meet. This not only affects the formation of emulsions in food industry but also impacts the cleaning efficiency of detergents and surfactants, prompting researchers and manufacturers alike to explore workarounds and innovative solutions.
Understanding Contact Angle
The contact angle, on the other hand, is a straightforward yet crucial measure of wetting or repelling behavior between a liquid and a solid. It is defined as the angle formed at the intersection of the liquid-air interface and the solid surface. This angle directly impacts how a liquid droplet behaves on a surface: it can either spread out thinly if the contact angle is small, indicating good wetting, or bead up if the angle is large, signifying poor wetting.
Let's consider a practical scenario: when applying a coat of paint. If the paint has a low contact angle on the surface, it means it will spread more evenly, resulting in a smooth finish. On the flip side, high contact angles can lead to uneven coats, affecting not just aesthetics but also durability. These interactions are important in areas like material science and manufacturing, where understanding and controlling these properties can enhance product development.
"In many cases, the efficacy of a system relies not just on the materials themselves but also on their interactions at the microscopic level."
Theoretical Background
Understanding the theoretical background of surface tension and contact angle is crucial to navigating the complexities of fluids and materials. This section forms a foundational stepping stone, linking the observed characteristics of liquids and solids to the underlying physical laws that govern their behavior. Grasping these principles not only sheds light on fundamental interactions but also provides the essentials for practical applications in various domains such as material science, biology, and engineering.
Physical Principles of Surface Tension
Surface tension arises from the cohesive forces between liquid molecules. Picture a cluster of water molecules near the surface; they behave differently than those submerged. The molecules at the interface experience greater inward forces as they are pulled by neighboring molecules, leading to a contraction of the surface. This phenomenon is not just a theory; it’s observable. For instance, look at how small insects can skim across a pond. Their ability rests on the high surface tension of water, a pool that acts almost like a taut skin.
There are vital forces at work here—specifically, molecular attraction and repulsion. As these forces interact, they dictate the shape that droplets take, often a sphere, as a means of minimizing surface area and energy.
It’s essential to recognize that surface tension can fluctuate based on temperature changes or the addition of surfactants, which disrupt the cohesive forces at the surface. Higher temperatures tend to lower surface tension because the kinetic energy of molecules increases, allowing them to break free from their neighbors more easily.
To fully comprehend the dynamics at play, scientists often express surface tension in terms of force per unit length (N/m). This quantitative measure aids researchers in determining how various materials will behave when in contact with a liquid.
Role of Surface Energy
Surface energy is a crucial concept that coincides with surface tension but focuses specifically on solids. This energy is essential for understanding how materials interact with liquids. When a liquid droplet makes contact with a solid surface, a battle ensues between the solid's surface energy and the surface tension of the liquid.
In simple terms, a high surface energy solid, like glass, will display different wetting properties compared to a low surface energy material, such as Teflon. In the case of glass, water tends to spread out, creating a low contact angle. Conversely, on Teflon, water beads up, increasing the contact angle. This behavior highlights how surface energy influences not just aesthetics but functional applications in coatings, sealing, and adhesion.
A practical take on this would involve applying these principles in industrial designs where a material's ability to resist wetting might be crucial—for instance, in non-stick cookware. Understanding these interactions allows engineers to create innovative designs that maximize or minimize wetting effects, based on the intended use.
"The significance of surface energy and its relation to surface tension cannot be overlooked; they dictate the compatibility of materials in real-world applications."
In summary, an appreciation of the physical principles of surface tension and the role of surface energy provides a comprehensive understanding of how liquids and solids interact. The implications of these concepts extend into everyday life, influencing product design, environmental science, and even biological systems.
Relationship Between Surface Tension and Contact Angle
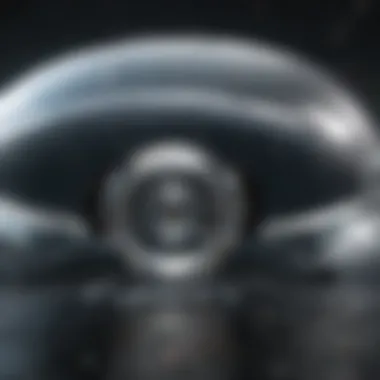
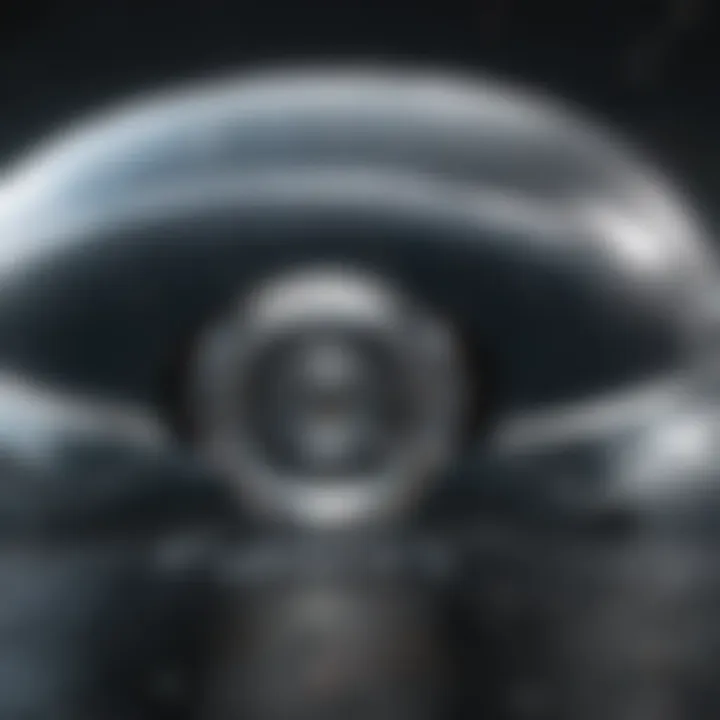
Understanding the connection between surface tension and contact angle is foundational in several scientific and engineering disciplines. This relationship not only influences material behavior but also heralds advancements in various technologies, such as coatings, adhesives, and biological systems. Essentially, surface tension can be thought of as the "skin" of liquids, driving how they interact with solid surfaces. Contact angle, on the other hand, describes the angle formed at the contact line where a liquid, solid, and gas phase meet, shedding light on the wetting characteristics of liquid substrates.
A key reason this topic is crucial is that it directly affects the efficiency of processes in industries ranging from pharmaceuticals to materials engineering. When scientists comprehend how surface tension pairs with contact angle, they can better design experiments, predict reactions, and optimize product formulations. For instance, a low contact angle could indicate a highly wettable surface, which may be desired for paints and coatings to achieve good adherence, whereas a high contact angle may be ideal for water-repellent fabrics.
Young's Equation: Foundation of the Calculation
Young's Equation stands as the cornerstone in outlining the relationship between surface tension and contact angle. The equation can be expressed as:
[\gamma_SV = \gamma_SL + \gamma_LV \cos(\theta) ]\
In this equation:
- (\gamma_SV) represents the solid-vapor interfacial tension,
- (\gamma_SL) denotes the solid-liquid interfacial tension,
- (\gamma_LV) is the liquid-vapor interfacial tension, and
- (\theta) signifies the contact angle formed.
The significance of Young's Equation lies in its ability to provide insights into how different materials interact with liquids. When you know the interfacial tensions, you can calculate the contact angle. This implication is big because it helps predict how changes in surface properties — like roughness or composition — will affect wetting behavior.
Influence of Substances on Contact Angle
Numerous substances can influence contact angles, affecting surface tension calculations. From surfactants to varying liquid compositions, the interactions can yield significant variations in the observed contact angle. For instance, adding surfactants to a solution will typically lower the surface tension, leading to a more pronounced wetting effect, shown with smaller contact angles. Conversely, impurities on a substrate can result in higher contact angles, decreasing the material's wettability.
Consider the case of a Teflon-coated surface. Teflon has a very low surface energy which results in high contact angles for water droplets, making it hydrophobic. This property is exploited in applications where resistance to water is critical. In sum, understanding how various substances alter the contact angle is essential for optimizing surface characteristics in practical applications.
This might raise some eyebrows in the scientific community, but as knowledge progresses, the analysis of how surface interactions vary with different substances ultimately leads to tailored solutions in product design and innovation.
Methods for Measuring Contact Angle
Understanding how to measure contact angles is pivotal in exploring the relationship between surface tension and contact angle. The accuracy of these measurements directly impacts any calculations that stem from them. Contact angle measurements reveal crucial information about the wetting characteristics of surfaces, influencing product design and material selection across various fields. Therefore, having reliable measurement methods provides a foundation to gain insights into behavior of fluids on solids.
Goniometry Techniques
Goniometry stands out as one of the most common methods for measuring contact angle. This technique involves utilizing a goniometer, which may sound technical but is essentially a camera and a protractor combined. The essence of measuring contact angle using goniometry lies in capturing images of a droplet resting on a solid surface.
Once the image is taken, the angles formed at the three-phase contact point—where the solid, liquid, and vapor interact—are determined. By calculating the angle formed between the tangent line at the liquid's surface and the solid surface, one is able to quantify the contact angle. Important to this method are considerations such as:
- Surface Preparation: Ensuring the test surface is clean and uniformly stable is crucial. Any contaminants can skew the results, leading to conclusions based on false data.
- Droplet Volume: Consistency in droplet volume is necessary. Whether it’s a larger droplet, which may spread more, or a smaller one, size variation can affect the apparent contact angle.
- Environmental Factors: Humidity and temperature at the moment of measurement can also influence the contact angle values. Performing measurements under controlled conditions helps in obtaining reproducible results.
Utilizing goniometric techniques is relatively straightforward, making it accessible even to those not specializing in surface science, reminding us that the simple tools can lead to profound insights.
Advanced Imaging Methods
As technology breaches new frontiers, advanced imaging methods have emerged, providing a more comprehensive view of contact angle measurements. Techniques such as high-speed imaging or digital image processing offer enhanced precision, opening a door to more sophisticated analysis of droplet behavior.
One significant method involves using a high-speed camera to capture rapid dynamics of droplet formation and spreading. For instance, this can illuminate how contact angle changes over time, rather than just providing a static measurement. Here are notable aspects of these methods:
- In-depth Analysis: Advanced imaging allows for tracking the droplet from its creation to its eventual resting form, providing insights into dynamic contact angles.
- 3D Imaging: Techniques like laser scanning and optical coherence tomography can create three-dimensional representations of the droplet and surface. This complexity offers a more robust understanding of interactions at the microscale.
- Software Models: With software solutions, researchers can analyze images in real time, allowing for a more nuanced understanding of how surfaces interact with various fluids.
"The future of contact angle analysis lies within its continuous evolution, especially with the integration of advanced imaging technologies."
In summary, using goniometry techniques provides a solid foundation for contact angle measurement, while advanced imaging methods elevate the complexity and depth of analysis. Understanding these methods not only enriches one’s comprehension of surface interactions but opens pathways for innovative research across varied fields.
Calculating Surface Tension from Contact Angle
Understanding how to derive surface tension through contact angle measurements is a crucial domain in material science and fluid dynamics. The relationship between these two phenomena offers a window into the interactions that govern the behavior of liquids and solids. By mastering this calculation, researchers and industry professionals can predict and manipulate how substances behave when mixed or layered, influencing everything from coating applications to biological materials.
Surface tension can best be viewed as an energetic barrier at the interface of a liquid and its surrounding. In contrast, the contact angle gives insight into how a liquid interacts with a solid surface—this is often defined by critical parameters such as cleanliness, roughness, and material properties, to name a few. The importance of this relationship cannot be overstated; it assists in, among other things, optimizing processes in chemical engineering and developing new materials with desirable wetting properties.
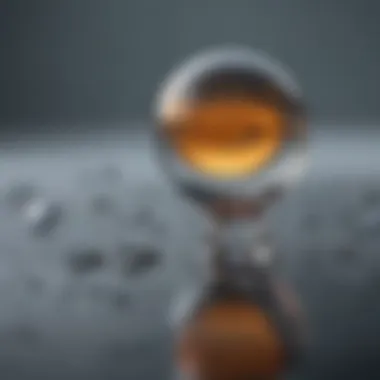
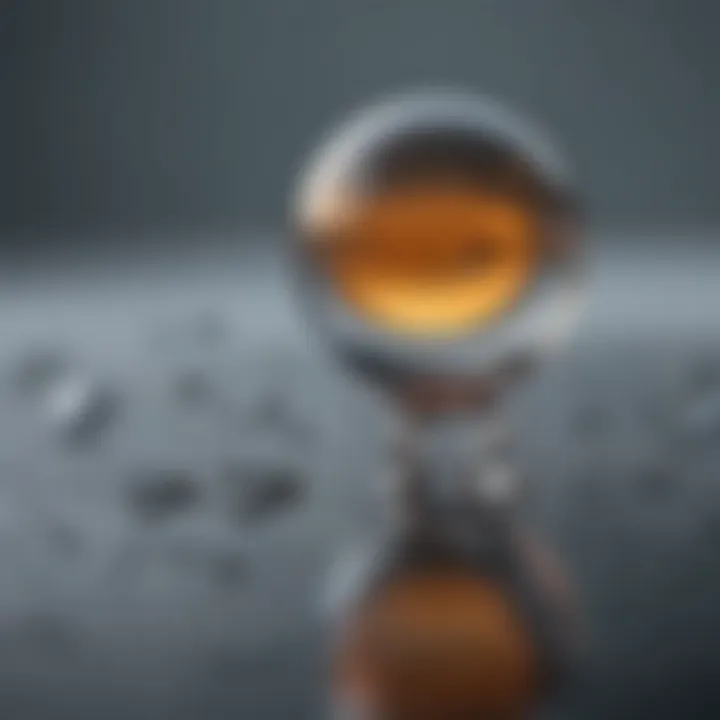
Key Benefits:
- Predictive power: By understanding how contact angle affects surface tension, professionals can better foresee how mixtures will behave in practical scenarios.
- Customized applications: Tailoring material surfaces for specific applications is facilitated by examining the surface tension that arises from contact angles.
- Research advancements: This relationship fuels innovative research across materials science and biophysics, enhancing our knowledge of interfacial phenomena.
Before diving deeper, let's clarify some foundational principles. This will help ensure that the calculations and methods discussed further down the line are grounded in a strong theoretical understanding.
Using Young's Equation
Young's Equation is foundational to calculating surface tension from contact angles. The equation itself is fairly straightforward, yet it encapsulates complex interactions that occur at an interface. It is expressed as:
[ \gamma_SV = \gamma_SL + \gamma_LV \cos(\theta) ]\
Where:
- ( \gamma_SV ) is the solid-vapor surface tension,
- ( \gamma_SL ) is the solid-liquid surface tension,
- ( \gamma_LV ) is the liquid-vapor surface tension,
- ( \theta ) is the contact angle.
This equation fundamentally illustrates how the balance between these surface tensions yields the contact angle observed, providing a pathway to determine either surface tension value when the others are known. It emphasizes the dynamic equilibrium at play—a slight alteration in any surface tension can cause significant changes in contact angle, further illustrating the sensitivity of these measurements to physical conditions.
In practice, measuring the contact angle accurately often requires meticulous setups and controls. An improvement in laboratory techniques, such as using high precision goniometers, can reduce errors in the measured angles and, consequently, improve the reliability of the calculated surface tensions.
Incorporation of Temperature and Pressure Effects
Surface tension and contact angles are not fixed values; they fluctuate notably with changes in temperature and pressure. This variability must be integrated when performing calculations.
Temperature effects can be explained via the molecular dynamics of a liquid. Generally, as temperature increases, the kinetic energy of the molecules rises, making them more mobile. This can lead to a reduction in liquid's surface tension, which can have cascading effects on how this layer interacts with solid materials. Therefore, in practical applications, one must consider how the changes in environmental temperature will influence the observed contact angles and calculated surface tensions.
Likewise, pressure can affect the physical state of materials and their interactions. High-pressure environments can lead to phenomena such as compression of liquid layers, potentially altering surface tensions. Understanding these relationships helps optimize processes such as coating technologies and material fabrication, ensuring desired interactions at the surface level.
In summary, calculating surface tension from contact angles is not simply a mechanical task; it's a sophisticated balancing act that must consider numerous physical factors. By melding theoretical frameworks like Young's equation with pragmatic measures of environment conditions, researchers can gain valuable insights into material behavior.
Applications of Surface Tension Calculations
Surface tension calculations don't just sit pretty in textbooks; they play a crucial role in the real world, especially in material science and biological contexts. By providing a quantitative measure of how liquids interact with solids, understanding surface tension can lead to groundbreaking advancements. Whether it’s developing new materials or enhancing medical applications, the implications are profound.
Material Science and Engineering
When diving into material science, surface tension isn't just a number to memorize. It's a key player in determining how materials behave when liquids are involved. For instance, in the production of coatings, understanding how well a liquid spreads on a surface can influence the effectiveness of that coating. If the surface tension is too high, the liquid may bead up rather than create a solid film, compromising protection against corrosion or other environmental elements.
In 3D printing, particularly for materials like polymers, surface tension calculations can help in optimizing the quality of printed objects. Variations in surface tension can lead to defects or inconsistencies in the final product, hindering the performance of manufactured structures.
Understanding surface tension is essential for tailoring material properties in real-world applications.
To put it simply, when engineers know the surface tension, they can fine-tune processes, ensuring better adhesion and integration of materials. This also plays a part in things like drug delivery systems, where how a liquid forms a droplet can impact how drugs are absorbed into systems.
Biological Systems and Medical Applications
Surface tension takes on another dimension in the realm of biology and medicine. Biological fluids, such as those found in our lungs, involve crucial interactions governed by surface tension. For example, surfactants in alveoli reduce surface tension to keep the lungs from collapsing, a vital process for proper respiration. Quantifying this can provide insights into various respiratory diseases and lead to targeted therapies.
In medical devices, understanding how fluids interact with surfaces is crucial. For instance, when blood comes into contact with the materials inside a catheter, surface tension influences how well a thrombus (clot) can form. Knowledge of these interactions allows engineers to design better, safer medical devices that mitigate complications.
In sum, the applications of surface tension calculations showcase not just the importance of theoretical knowledge, but its far-reaching impact in practical scenarios. So, whether it's enhancing materials or revolutionizing medical practices, the understanding of surface tension is paramount.
Limitations and Considerations
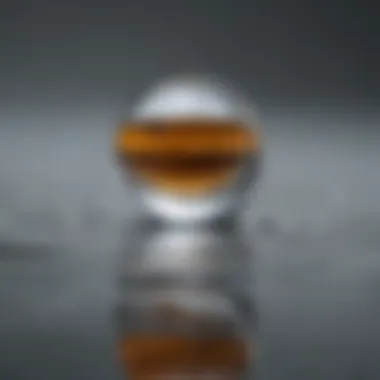
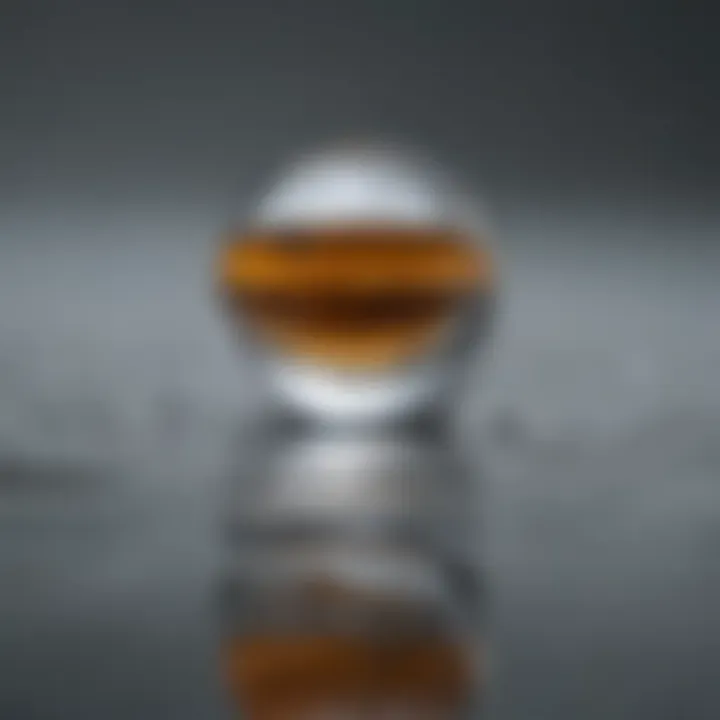
When embarking on the journey of calculating surface tension through contact angles, one must not overlook the limitations and considerations inherent in this method. Understanding these aspects is crucial not only for accurate calculations but also for meaningful interpretations of the data. This section delves into two primary considerations: the challenges posed during measurement and the influence of surface contaminations, both of which can significantly impact the reliability of the results.
Challenges in Measurement
Measuring contact angles requires precision and control. Many factors come into play that can skew the results, rendering a professional's best efforts potentially futile. Some of the key challenges include:
- Environmental Conditions: Variations in temperature and humidity can cause significant deviations in measurements. A small change in room temperature might lead to a noticeable difference in the contact angle, leading to erroneous conclusions about surface tension.
- Instrument Calibration: Devices used to measure contact angles, such as goniometers, must be meticulously calibrated. If the calibration is off, the data collected could mislead researchers regarding the solvent interactions and properties.
- Surface Roughness: An element often overlooked is the texture of the material's surface. Roughness can trap liquid, leading to enhanced or diminished contact angles that aren’t indicative of the true surface tension.
It's vital for researchers to remain cognizant of these challenges and to develop robust methodologies to mitigate them. Repeating experiments under controlled conditions or utilizing standardized procedures can help to ensure data accuracy.
Impact of Surface Contaminations
Surface contaminations, whether they originate from environmental sources or during sample preparation, can exert substantial effects on the measurements taken. Common types of contaminations include:
- Dust and Particulates: Even minuscule particles on a surface can obstruct the true contact angle measurement, leading to inflated or deflated values. For instance, a particle that disrupts the liquid droplet can interfere with the expected meniscus shape.
- Chemical Films: Residues from previously used substances might form a layer on the surface that competes with the liquid for adhesion. This affects the contact angle observed and therefore the calculated surface tension.
- Moisture: Water vapour in the environment can condense on the surface, leading to complications in defining what one is measuring. It’s often necessary to dry the sample thoroughly to negate this effect.
By directly addressing these contaminants, researchers can enhance the reliability of their measurements. Implementing cleaning protocols and maintaining a controlled environment can significantly diminish the influence of these factors.
Future Perspectives
Exploring the future perspectives of surface tension and contact angle research is akin to looking through a window into a world ripe with potential. The methodologies and knowledge we have discussed not only advance our current practices but also pave the way for new techniques that can enhance various fields. Understanding these future directions holds paramount importance because it helps students, researchers, educators, and professionals stay ahead of the curve in a constantly evolving scientific landscape.
New advancements are cropping up in surface science, showing how crucial it is to stay updated. The synthesis of innovative materials, for instance, is an area where accurate measurements of surface tension and contact angles will play a role in designing surfaces with specific desired properties. The increased interest in nanotechnology also highlights the significance of these calculations, as the behavior of materials at the nanoscale often deviates from traditional observations.
Evolving Techniques in Surface Science
With each passing year, new techniques emerge, challenging the old guard and offering fresh insights into previously complicated concepts. Take the advent of microfluidic devices, for example. These technologies not only allow for precise control of fluid movement at the microscale but also enable the examination of surface tension under varied conditions.
An array of cutting-edge imaging techniques is also gaining traction. Techniques such as atomic force microscopy (AFM) and scanning electron microscopy (SEM) are not just enhancing our understanding of material surfaces, but are also revolutionizing the way contact angles are measured. The precision these methods offer could push the boundaries of quantitative analysis in surface science.
Moreover, the integration of artificial intelligence in evaluating contact angles presents another realm of possibilities. Machine learning algorithms can potentially analyze vast datasets far quicker than human analysis, leading to real-time insights into surface interactions and enabling rapid assessments of changes in material properties.
Interdisciplinary Approaches
The beauty of research in surface tension and contact angles lies in its interdisciplinary nature. As the lines continue to blur between various scientific domains, fostering collaboration among chemists, physicists, material scientists, and engineers becomes increasingly important. Such interdisciplinary approaches can drive innovations that may not have been possible within the confines of conventional specializations.
For instance, a cross-disciplinary exploration of biological systems can provide insights into how surface tension affects cellular interactions and drug delivery mechanisms. By blending insights from biology and surface science, novel therapeutics could emerge that harness these principles to enhance efficacy.
Moreover, environmental science also stands to gain from a deeper understanding of these phenomena. As we grapple with pressing issues like pollution, an interdisciplinary angle could spotlight how contact angles affect surface interactions in real-world scenarios, leading to effective solutions.
In summation, focusing on future perspectives, new techniques, and broadening our horizons through interdisciplinary collaboration are essential for progressing our understanding of surface tension and contact angles. Each stride in this field has the potential not only to refine current methodologies but also to uncover new applications that can significantly impact diverse areas ranging from materials science to environmental health.
"Innovation is often born at the intersection of fields, where different ideas meet and merge."
Staying attuned to these advancements will not only fortify our foundational knowledge but also enable us to harness the full potential of surface interactions in whatever application lies ahead.
Ending
In wrapping up our exploration into the interplay of surface tension and contact angle, we must take a moment to clarify the pivotal role these concepts hold in both theoretical and practical realms. Understanding how to calculate surface tension from contact angles not only serves to bolster scientific inquiry but also finds significance across various applications.
Summarizing Key Points
To summarize, here are the essential elements we’ve covered in the article:
- Foundation of Surface Tension and Contact Angle: We have defined these fundamental concepts and their significance in fluid dynamics and material science.
- Young's Equation: This equation forms the backbone of our calculations, bridging surface tension with contact angles across solid-liquid interfaces.
- Measurement Techniques: We’ve discussed methods such as goniometry and advanced imaging that facilitate accurate measurement of contact angles, leading to precise surface tension calculations.
- Applications: Surface tension calculations are critical not just in academic research but also in industrial applications, influencing product design, coatings, and even biological systems.
- Challenges: We’ve delved into the limitations faced in measurement, like sample preparation and environmental contamination, which can skew results.
The Importance of Accurate Calculations
Accuracy in these calculations is paramount. The reliance on precise measurements cannot be overstated, as the consequences of inaccuracies can ripple through various fields. For instance, in material science, selecting the right materials for specific applications often hinges on understanding their surface properties. Without accurate calculations, one risks making misinformed decisions that could lead to failures or subpar performance in final products. Additionally, in biomedical applications, where surface interactions can directly influence biological responses, precise calculations help inform the design of implants, drug delivery systems, or diagnostic tools. Simply put, cultivating accuracy not only enhances scientific understanding but also protects the integrity of applied practices.
"In science, precision isn’t just a luxury; it’s an obligation."