Revolutionizing Science: Breakthroughs in Microscopy
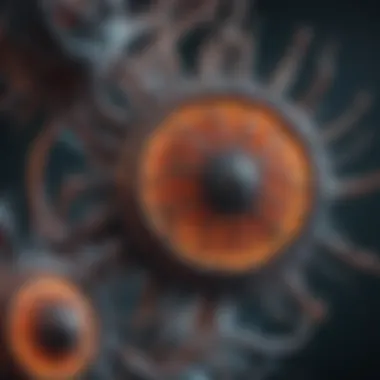
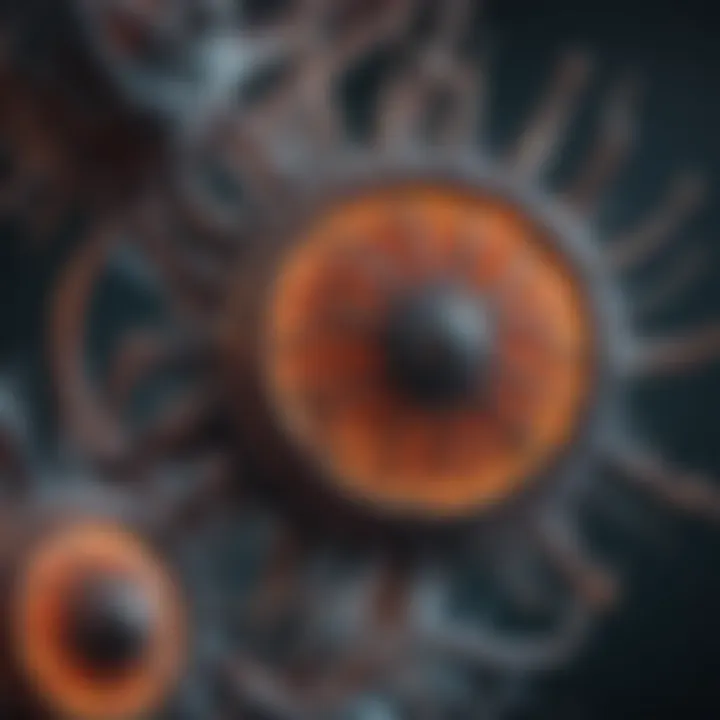
Intro
Microscopy has always played a vital role in the pursuit of scientific knowledge. Advances in this field have allowed researchers to explore the unseen, revealing secrets that were previously thought unreachable. Its evolution reflects not only technological progress but also the growth of our understanding of biology, materials science, and beyond. This article presents an engaging and detailed examination of how modern innovations are reshaping microscopy, elevating it beyond its original applications and unlocking new frontiers in research and discovery.
Research Overview
Summary of Key Findings
Recent advancements in microscopy have introduced novel techniques and technologies that extend far past traditional microscopy methods. For instance, the development of super-resolution microscopy has enhanced our capability to visualize cellular structures at unprecedented resolutions. Furthermore, the integration of artificial intelligence in imaging analysis has increased the accuracy of results, making microscopy more efficient and powerful.
The implications of these advancements affect multiple scientific fields, including biology, materials science, and nanotechnology. Researchers are witnessing a new era where previously undetectable phenomena can now be observed and studied comprehensively.
Research Objectives and Hypotheses
The key objectives of this discourse include:
- To delineate how advancements in microscopy contribute to the scientific landscape.
- To critically analyze emerging technologies that enhance imaging capabilities.
- To underscore the broader implications of microscopy innovations across varied research domains.
The underlying hypotheses posited in this research suggest that continued investment and exploration in microscopy will not only foster new discoveries but also encourage interdisciplinary collaboration, inspiring further technological advancements and scientific inquiry.
Methodology
Study Design and Approach
This article employs a qualitative approach to systematically examine the state of microscopy today. Existing literature, case studies, and technological reviews are analyzed to frame our understanding and to highlight the trajectory of microscopy innovations. By synthesizing various sources, we gain a comprehensive perspective of the current landscape.
Data Collection Techniques
Data for this research is collected from numerous reputable sources. Literature reviews, empirical studies, and expert opinions from various scientific journals are utilized. This approach enables a broad yet detailed viewpoint, capturing advancements that may otherwise be overlooked in a singular narrative.
"The power of microscopy lies not just in what we can see, but in what we can learn from those tiny details."
Throughout the coming sections, the article will continue to delve deeper into specific technologies and their ramifications, effectively linking the progression of microscopy to the larger canvas of scientific discovery.
Intro to Microscopy
Microscopy stands as a cornerstone in the realm of scientific inquiry. It bridges the gap between the visible world and the minutiae of life form that remain hidden to the naked eye. The ability to see structures at a microscopic level, in turn, elevates our understanding of biology, materials science, and a multitude of other disciplines. This section will introduce the fundamental aspects of microscopy, revealing its significance and the insights it provides into both living systems and synthetic materials.
Definition of Microscopy
Microscopy refers to the technique of using various instruments and methodologies to view objects and structures too small to be seen without enlargement. It encompasses a range of imaging methods that have evolved from simple optical scopes to complex imaging systems that utilize electrons and other advanced technologies. The essence of microscopy lies in its capacity to transform our perception of the minute, broadening our understanding and facilitating discoveries that would otherwise remain obscured.
Historical Evolution of Microscopy
The journey of microscopy is rich and layered, marked by groundbreaking milestones that have expanded our scientific field.
Early Discoveries in Microscopy
The initial foray into microscopy can be traced back to the late 16th century, with pioneers such as Hans Janssen and his son Zacharias, who experimented with lenses. Their formulation of the compound microscope was revolutionary. This technique allowed for magnified views of tiny specimens, uncovering intricate biological structures that had remained uncharted. Such early microscopes were rudimentary yet set the foundation for further exploration. They proved instrumental in opening discussions around cellular structures, setting the stage for the cell theory.
The main characteristic of these early microscopes was their simplicity. They were not equipped with advanced optics but relied on basic magnification principles. This simplicity, however, also meant they had significant limitations regarding resolution and clarity, affecting the quality of insights obtainable from observations.
Advancements in Optical Lenses
The evolution of microscopy was accelerated by significant advancements in optical lenses. The 19th century heralded new lens-making techniques, improving light transmission and enhancing resolution. Figures like Joseph Jackson Lister and Carl Zeiss were pivotal in this transformation. They discovered methods to reduce optical aberrations, producing clearer and more precise images.
These advancements brought microscopy into laboratories around the world. By utilizing high-quality glass and precision grinding, researchers could now observe microstructures in finer detail. Improved lenses played a critical role in advancing scientific knowledge, particularly in biology, where the ability to observe cells in greater detail unlocked numerous avenues for research.
The unique feature of these advancements ensures greater applicability in various scientific fields. While they enhanced the practicality of microscopy, they also required increased knowledge and skill from users to fully leverage the sophisticated technology available. Therefore, while beneficial, the increased complexity also introduced challenges in the standardization of practices across different laboratories.
In summary, the historical evolution of microscopy illustrates a narrative of innovation and relentless pursuit of knowledge. Each development has contributed invaluable tools and insights to our understanding of the microscopic world.
The Foundation of Microscope Technology
The foundation of microscope technology is critical as it shapes our understanding and utilization of microscopic imaging. Without a solid foundation in microscope technology, advancements in scientific research would be severely limited. This section aims to elucidate the various types of microscopes as well as the key components integral to their function. By examining these elements, we can appreciate how they serve as the backbone of modern microscopy. Their importance is not just historical; it influences contemporary research and applications across numerous disciplines.
Types of Microscopes
Optical Microscopes
Optical microscopes are among the most commonly used types of microscopes. They function by utilizing visible light and a system of lenses to magnify samples. One key characteristic of optical microscopes is their ease of use and accessibility. For educational settings and routine laboratory work, they often serve as the preferred choice for students and researchers alike.
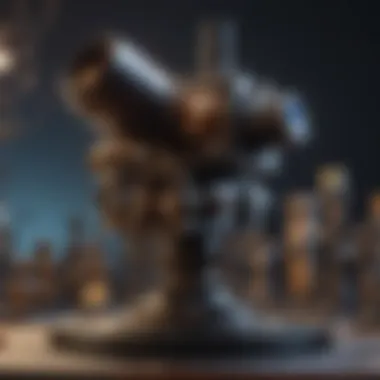
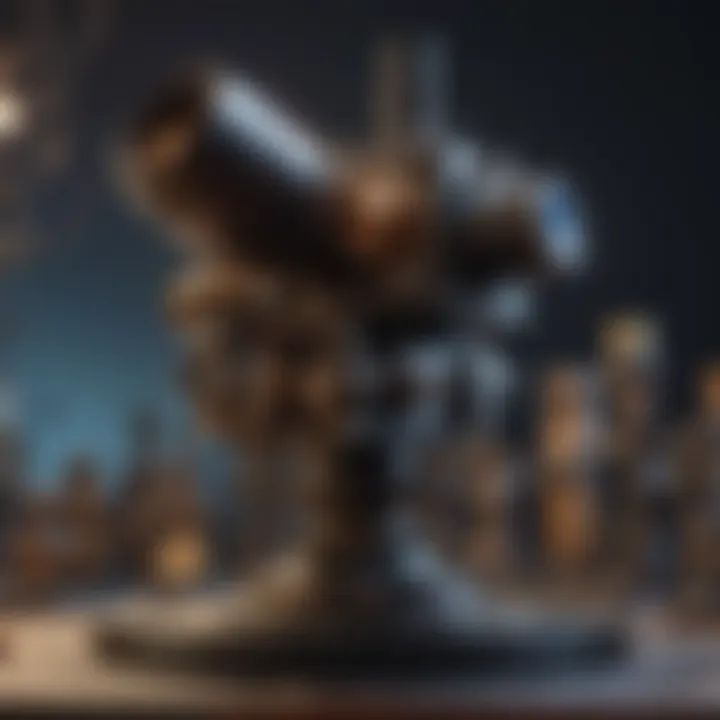
The unique feature of optical microscopes is that they can provide real-time images, allowing for observations of live specimen. This aspect is particularly beneficial in biological studies where immediate response observations are necessary. However, they are limited in resolution and are not capable of visualizing structures smaller than the wavelength of light, which can be a disadvantage in certain high-resolution applications.
Electron Microscopes
Electron microscopes represent a significant advancement in imaging technology. They use electron beams instead of visible light to illuminate samples, which allows for much greater magnification and detail. One of the main advantages of electron microscopes is their ability to achieve resolutions that are orders of magnitude higher than optical microscopes. This capability makes them essential in fields like materials science and cell biology.
Their unique feature lies in the ability to visualize the fine details of structures at the atomic level. However, the use of electron microscopes comes with drawbacks, such as the high cost of equipment and the requirement that specimens be prepared in ways that often kill the samples. This can limit the scopes' applications in live cell imaging.
Scanning Probe Microscopes
Scanning probe microscopes are another essential type of microscopy technology. Unlike optical or electron microscopes, scanning probe microscopes do not rely on lenses or light, but instead use a physical probe to scan the surface of a sample. This innovative approach allows them to achieve atomic resolution.
A key characteristic of scanning probe microscopes is their versatility. They can be used to image a wide range of materials, making them valuable in many scientific fields. The unique feature of these microscopes is their capability to manipulate and even modify materials at the atomic level, which opens up new possibilities in nanotechnology. However, their complexity and the necessity for a controlled environment can be drawbacks when compared to other types of microscopes.
Key Components of a Microscope
Lenses and Objectives
Lenses and objectives are fundamental components of microscopes, as they are crucial for magnifying samples. Lenses bend light rays to form images, with objectives being specific types of lenses that determine the resolution and contrast of the image produced.
The key characteristic of lenses and objectives is their ability to focus light at various magnifications. This makes them indispensable for different levels of research, ranging from hobbyists to advanced scientific investigations. A unique aspect of these lenses is their numerical aperture, which influences how much light enters the lens and defines the resolving power of the microscope.
However, certain limitations exist, as objectives can be sensitive to alignment and contamination, which can affect imaging quality.
Illumination Systems
The illumination system is critical because it impacts image quality and visibility. Different types of illumination systems, such as transmitted and reflected light systems, offer unique advantages depending on the specimen being observed.
One key characteristic of illumination systems is their capacity to enhance contrast, enabling clearer images. Additionally, advanced systems like fluorescence illumination contribute significantly to the visualization of specific components within samples. However, the setup of these systems can be complex, often requiring trained personnel for optimal use.
Sample Stages
Sample stages are where specimens are placed for observation, and they play an integral role in microscopy. A stable and adjustable sample stage allows researchers to manipulate samples, providing the capability to analyze them from various angles.
The key characteristic of sample stages is their ability to hold specimens securely while allowing for precise movements. They are a crucial component in ensuring that researchers can obtain accurate images without introducing motion artifacts. One unique feature is the motorized stages available for high-precision applications, though these are often more expensive and require additional setup.
"A well-functioning microscope is only as good as its components, each playing a vital role in the imaging process."
The foundation of microscope technology presents a comprehensive overview of the significant types and components that shape the field. Understanding these elements enables a deeper appreciation of how advancements in microscopy continue to push boundaries in scientific exploration.
Innovative Techniques in Microscopy
Innovative techniques in microscopy play a critical role in advancing the field of scientific research. They extend the resolution and capabilities of standard microscopy methods, enabling scientists to observe and analyze structures at unprecedented levels. The integration of these techniques improves diagnostic precision and expands potential applications across diverse fields, particularly in biological and material sciences.
Fluorescence Microscopy
Fluorescence microscopy has revolutionized imaging techniques in the life sciences. It relies on the emission of light by a fluorophore, which is excited by a specific wavelength. This technique enhances contrast and provides clear images of structures on a cellular level. The key characteristic of fluorescence microscopy is its ability to label specific components within a sample, allowing for targeted visualization of different cellular components. The versatility of fluorescent markers makes this method a favored choice for researchers, particularly in cellular biology.
- Unique Feature: The capacity to utilize multiple fluorescent markers simultaneously allows for multi-parameter analysis.
- Advantages: High sensitivity and specificity in localization of proteins, nucleic acids, and other biomolecules. However, a potential disadvantage includes photobleaching, where repeated exposure to excitation light can degrade the fluorescent signal over time.
Applications in Biological Research
Applications in biological research emphasize the significance of fluorescence microscopy in various subfields, such as developmental biology and neurobiology. This technique supports the investigation of complex structures at high resolution, impacting our understanding of cellular processes.
- Key Characteristic: Fluorescence microscopy enhances our capacity to study dynamic processes in live cells, which is vital for real-time observations.
- Unique Feature: Real-time imaging reveals intricate cellular interactions and functions over time. The downside might include the cost and availability of high-quality fluorescent dyes and markers, which could limit some studies.
Super-resolution Microscopy
Super-resolution microscopy takes imaging a step further by surpassing the diffraction limit of light, which traditionally restricted resolution. Techniques such as STED (Stimulated Emission Depletion) and PALM (Photo-Activated Localization Microscopy) enable researchers to visualize structures at a nanoscale level. The key characteristic of super-resolution techniques is their ability to provide detailed insights into cellular architecture, which is crucial in understanding complex biological phenomena.
- Unique Feature: The potential to achieve resolutions down to tens of nanometers.
- Advantages: Enables the observation of molecular distributions and interactions with higher fidelity than conventional methods. Nonetheless, these methods often require complex sample preparation and specific imaging conditions.
Impacts on Cellular Biology
The impacts of super-resolution microscopy on cellular biology are profound. By allowing scientists to visualize cellular components with unprecedented detail, these techniques open doors to insightful discoveries regarding cellular behavior and mechanisms.
- Key Characteristic: The ability to study live cells with high spatial resolution.
- Unique Feature: The technique enhances our understanding of the localization and dynamics of proteins in living organisms, revealing important aspects of cell signaling and disease progression. However, the technical complexity involved may pose challenges for routine use in certain labs.
Cutting-Edge Microscopy Methods
Cutting-edge microscopy methods represent significant advancements in how researchers visualize and analyze the microscopic world. These methods push the boundaries of traditional microscopy, enabling the observation of structures and processes at unprecedented resolutions. They provide vital insights across various scientific disciplines, thereby enhancing our understanding of complex biological systems and materials.
Cryo-Electron Microscopy
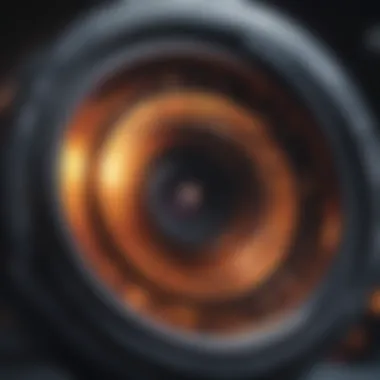
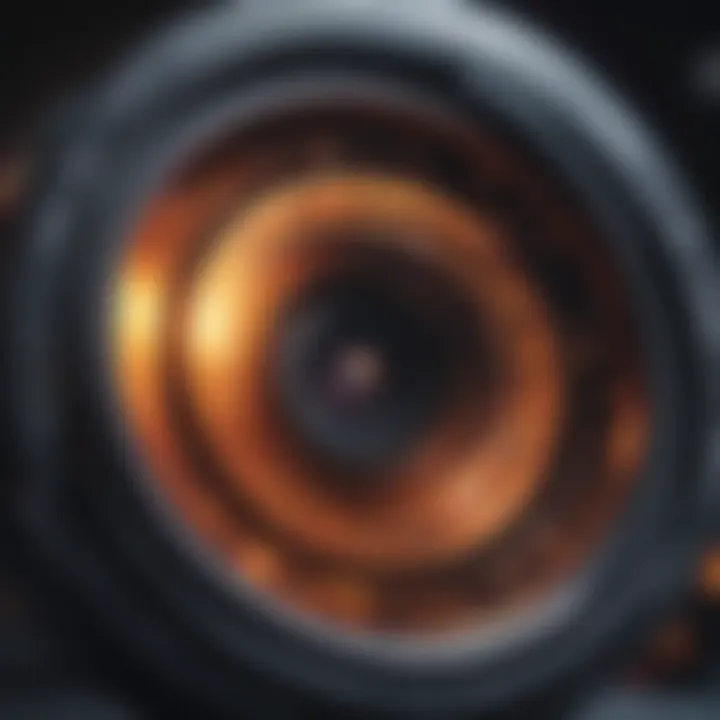
Principles of Cryo-EM
Cryo-electron microscopy, commonly referred to as Cryo-EM, involves the rapid freezing of biological samples that preserves their natural structure. This approach avoids the damaging effects associated with standard imaging techniques. A key characteristic of Cryo-EM is its ability to visualize samples at near-atomic resolution. This resolution enables scientists to study intricate details of macromolecules and their interactions.
The unique feature of Cryo-EM lies in its adaptability to various samples, from viruses to protein complexes. The primary advantage of this technique is its capability to provide high-resolution images without the need for extensive staining or fixing that can alter the natural state of biological specimens. However, a potential disadvantage is the complexity and cost of equipment, which may limit access in some research settings.
Revolutionizing Structural Biology
The impact of Cryo-EM in structural biology cannot be overstated. This method is revolutionizing our approach to understanding how biological macromolecules function. By providing detailed insights into the three-dimensional structures of proteins and other biomolecules, Cryo-EM enables researchers to formulate hypotheses related to mechanisms of action, interactions, and functions at a molecular level.
This technique's significance is highlighted by its rapid acceptance and application in numerous high-profile studies, including those focused on membrane proteins and complex cellular structures. A defining feature is the ability to visualize samples in a near-native state, making it particularly beneficial for studying dynamic processes. However, some limitations include the need for specialized training to obtain and interpret the data adequately, which can constrain its widespread use.
Nano-Optics in Microscopy
Emergence of Nano-optical Techniques
Nano-optics represents a pivotal advancement in microscopy, utilizing principles of optics to observe materials at the nanoscale. These techniques allow scientists to overcome the diffraction limit encountered in traditional optical microscopy. A key characteristic of nano-optical techniques is their ability to manipulate light at sub-wavelength scales, providing enhanced imaging capabilities.
The unique feature of these techniques is their utilization of phenomena such as plasmonics, which enables visualization of structures that are otherwise impossible to detect. One major advantage of nano-optics is the capability of achieving high spatial resolution, resulting in detailed images of nanoscale features in various materials. Conversely, the complexity of these setups and the necessity for sophisticated data analysis can present challenges for researchers.
Applications in Material Science
In material science, the applications of nano-optics are vast and diverse. They enable detailed examination of nanomaterials, assessing properties such as conductivity and refractive index. An important characteristic of these applications is the ability to study the behavior of materials under various conditions, including stress and temperature changes.
This capability is a significant benefit that supports innovations in fields like nanotechnology and electronics. The unique feature of using nano-optical methods is that they can yield real-time data, contributing to faster advancements in material development. However, the equipment and expertise required for these methods may limit accessibility in some contexts, impacting how broadly they can be applied in research.
The Role of Artificial Intelligence in Microscopy
The integration of artificial intelligence (AI) in microscopy represents a crucial leap forward in the field. AI technologies have the capacity to manage large volumes of complex data generated by advanced microscopy methods. The significance of AI lies in its ability to improve both the efficiency and accuracy of image processing and interpretation. Moreover, these algorithms can discover patterns that might be invisible to the naked eye. Thus, AI is not merely a tool for analysis but a transformative element redefining how microscopy data is understood and leveraged across scientific research.
AI Algorithms in Image Processing
AI algorithms enhance the process of image analysis in microscopy in multiple ways. These algorithms can optimize image clarity, reduce noise, and improve resolution. Most commonly, tools such as convolutional neural networks (CNNs) assist in classifying and segmenting specimen images rapidly. This technology allows researchers to analyze more samples in less time, substantially accelerating the pace of study.
- Benefits of AI in image processing include:
- Increased accuracy in identifying structures.
- Automation that minimizes human error.
- Rapid processing of extensive datasets.
AI algorithms can also facilitate real-time analysis. This capability is particularly valuable in fields like biomedical research, where timely insight can lead to breakthroughs in understanding diseases.
Enhancing Analysis and Interpretation
The impact of AI on the analysis and interpretation of microscope images extends far beyond mere automation. AI empowers scientists by providing tools for predictive analysis and advanced data mining. For example, machine learning models can be trained to predict outcomes based on historical data, which can lead to new hypotheses and innovative approaches in various studies.
Benefits of enhancing analysis through AI include:
- Greater precision in diagnostic techniques.
- Improved identification of anomalies in biological samples.
- Insights into complex interactions within cells and tissues.
Through these systems, researchers can uncover intricate details about biological processes that were previously difficult to resolve. This synergy between AI and microscopy signifies not just an evolution but a revolution in how microscopic data is harnessed for scientific advancement.
The computational power of AI allows for a new level of interpretation that supports researchers in making informed decisions in their investigations. As AI technologies continue to evolve, their role in microscopy is expected to deepen, offering even more promising applications.
Applications of Modern Microscopy
The application of modern microscopy has become a core element of contemporary research and innovation. It extends beyond mere observation to significantly influence various fields including biology, materials science, and engineering. The advancements in microscopy techniques allow scientists and researchers to uncover the invisible structures and dynamics at the micro and nano scales. This access leads to new understanding and applications that were not possible with earlier technologies.
Biomedical Applications
Biomedical applications of microscopy are critical for advancing our knowledge of biological systems. The ability to visualize cellular processes in real time provides insights into how organisms function and respond to various stimuli. Here are two significant subfields within biomedical applications:
Cell Imaging and Tracking
Cell imaging and tracking is crucial for understanding the life cycles of cells. This technique allows researchers to observe how cells grow, divide, and interact with one another. The use of fluorescence microscopy enhances this research. It enables scientists to use specific dyes that label certain cell components, making them visible under the microscope. A key advantage of this approach is its capacity to track the movement of individual cells over time, revealing patterns that can inform about disease progression or treatment efficacy.
However, there are challenges in ensuring that the fluorescent markers do not interfere with cellular functions. This aspect requires careful consideration to prevent the distortions in observed results.
Drug Discovery and Development
Drug discovery and development heavily rely on modern microscopy techniques. These methodologies facilitate the visualization of how potential drugs interact with cells and tissues. Advanced imaging methods, such as confocal and high-content screening, enable researchers to evaluate drug effects at multiple cellular levels. One key characteristic is the detailed information obtained about drug localization and mechanisms of action within living cells. This provides valuable insights that can direct the design of more effective therapeutics.
The unique feature of this approach is its ability to analyze thousands of specimens simultaneously, significantly speeding up the drug development process. However, the complexity of data analysis can be a disadvantage, requiring sophisticated algorithms and computational resources to derive meaningful conclusions.
Materials Science Applications
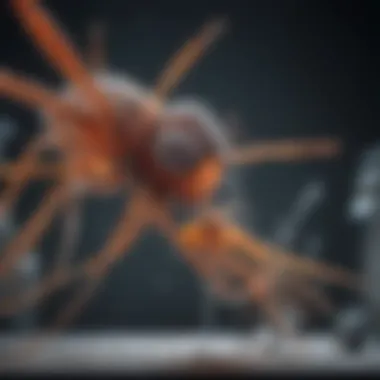
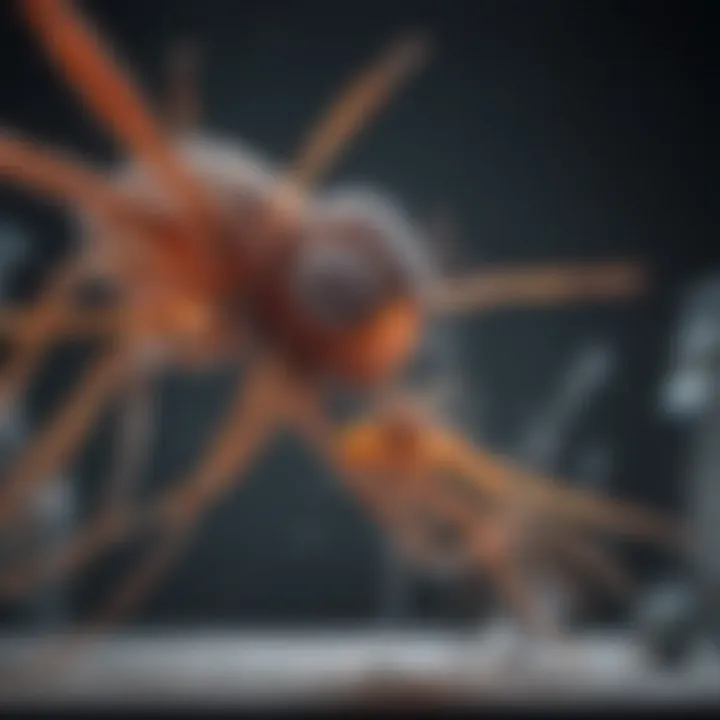
Material science applications greatly benefit from advancements in microscopy. Understanding materials at the nanoscale opens new frontiers in technology and engineering. The following subsections detail two important areas of focus:
Nanomaterials Characterization
Nanomaterials characterization is a vital process for developing advanced materials with unique properties. High-resolution techniques like scanning electron microscopy (SEM) and atomic force microscopy (AFM) provide insights into material surface structures and compositions. These techniques allow scientists to tailor materials for specific applications by understanding their physical and chemical properties at the nanoscale.
A significant advantage of nanomaterials characterization is its ability to provide precise measurements and visualizations of structures that influence material properties. Yet, challenges remain regarding the reproducibility of measurements and scalability of techniques for industrial applications.
Failure Analysis in Engineering
Failure analysis in engineering is critical to understanding why materials or components fail during use. Microscopy techniques enable detailed examination of failed components, helping to identify root causes of failure. This could involve scrutinizing fracture surfaces or analyzing material degradation processes.
A key characteristic of failure analysis is its role in enhancing safety and reliability in engineering design. By understanding failure modes, engineers can innovate safer materials and structures. Nonetheless, the time-consuming nature of some microscopy methods can delay product improvements or redesigns.
Ethical Considerations in Microscopy Research
As microscopy technology advances, ethical considerations become increasingly significant. The ability to visualize the microscopic world opens new avenues for discovery but also raises important moral and ethical issues. This section discusses key elements that shape the ethical landscape in microscopy research, focusing on data integrity, reproducibility, and the implications of these factors on society.
Data Integrity and Reproducibility
Data integrity refers to the accuracy and consistency of data collected during microscopy research. High standards for data management are crucial, especially in a field where findings can influence future research, treatments, and policy decisions. Ensuring data integrity involves maintaining detailed records, utilizing standardized procedures, and employing rigorous validation methods.
Reproducibility is closely linked to data integrity. It is critical that other researchers can replicate experimental results to confirm findings. There are many factors that affect reproducibility, including variations in sample processing, imaging settings, and analysis techniques. When researchers share their methodologies transparently, it fosters trust and may speed up the advancement of science.
When ethical breaches occur, such as data fabrication or misleading representations of results, the scientific community suffers. Such actions undermines public trust and can halt advancements in medicine, biology, and various other fields that rely on microscopy. To mitigate these risks, the implementation of strict ethical guidelines and fostering an open culture of sharing methods and results are necessary steps.
Implications of Microscopy on Society
The implications of microscopy go beyond scientific discovery; they touch upon societal values and ethical standards. Microscopy technology can lead to breakthroughs that affect public health, environmental monitoring, and material sciences. However, it also raises critical questions surrounding privacy and consent, particularly in biomedical research.
For example, the use of microscopy in studying human tissues demands clear guidelines on how samples are collected, who has access to this data, and how it is used. Ethical frameworks must protect individual rights while allowing for scientific progress. Missteps in these areas can result in harm to individuals and communities.
Moreover, findings derived from microscopy often influence public policy. As scrutiny on scientific data increases, it is essential that data is not only reproducible but also ethically sourced and interpreted. Given the stakes involved, researchers must engage with this ethical discourse actively and develop frameworks that address the complex intersections of science, ethics, and society.
"The trustworthiness of scientific engagement is contingent upon unimpeachable ethical practices amid advancing technologies."
In summary, ethical considerations in microscopy are pivotal. They guide researchers in maintaining high standards of integrity and reproducibility, ensuring that the advancement of science remains beneficial to society. As technology progresses, a robust ethical framework must evolve simultaneously, safeguarding public interest while fostering innovation.
Future Perspectives in Microscopy
The realm of microscopy is on the brink of radical transformation. As scientific challenges evolve, the advances in technology herald a new age of exploration and understanding. This section elaborates on future perspectives in microscopy, not only highlighting the expected innovations but also emphasizing the importance of interdisciplinary collaboration. Such advancements hold the promise to reshape the way researchers view and interpret data at microscopic levels.
Expected Innovations
Innovation in microscopy will likely stem from various technological breakthroughs. Some anticipated innovations include:
- Enhanced Imaging Resolution: Continuing to push beyond current limits, new techniques may allow researchers to capture images with superior clarity.
- In Situ Analysis: The ability to observe biological processes in real-time within their natural context will facilitate dynamic studies.
- Integration of AI and Machine Learning: Utilizing artificial intelligence can dramatically improve image analysis, allowing for faster and more accurate interpretation of complex datasets.
- Smart Imaging Systems: Future microscopes could employ automation to adjust settings autonomously based on sample characteristics, significantly improving user experience and research efficiency.
These innovations promise to broaden the horizons of microscopy. For instance, improved resolution can unveil previously undetectable cellular components, leading to insights into cell functionality and disease processes.
Integration of Multidisciplinary Approaches
The interdisciplinary approach is key to future advancements in microscopy. Collaboration among fields such as biology, physics, computer science, and engineering is essential. By merging expertise from various disciplines, researchers can tackle complex scientific questions more effectively.
- Biologists can provide insights into necessary biological markers and relevant processes.
- Physicists can lend their knowledge on optics and imaging techniques, fostering new methods to manipulate light.
- Computer scientists can develop algorithms that enhance image processing and data analysis, streamlining research workflows.
- Engineers can focus on engineering better instruments and automating processes, which leads to higher efficiency and precision.
Through this synergistic approach, the future of microscopy is not just about technological advancements. It involves a broader understanding of how these tools can be employed to answer pressing scientific inquiries. This collaborative spirit will likely enhance the analytical capacity of researchers, leading to innovative solutions and discoveries.
The End
The conclusion serves as a pivotal section of the article, encapsulating the essence of significant advancements in microscopy. It synthesizes crucial insights that have emerged throughout the discourse, drawing a comprehensive picture of how microscopy has evolved over the years. Recognizing the transformative impact of this technology is essential to understand its far-reaching implications on science and society. The advancements not only enhance our viewing capabilities but also facilitate groundbreaking research across multiple fields, from materials science to biological studies.
Summary of Key Insights
In this article, several major points are highlighted:
- Historical Context: The journey of microscopy from its rudimentary beginnings to present-day high-resolution techniques.
- Innovative Techniques: Introduction of methods like fluorescence and super-resolution microscopy that move beyond traditional imaging.
- Role of AI: How artificial intelligence streamlines image analysis and interpretation, furthering the potential of microscopy in research.
- Ethical Considerations: Awareness of data integrity and societal impacts surrounding microscopy research.
- Future Directions: The expected innovations and the integration of diverse scientific approaches to push boundaries further.
These insights reflect the synergy between technology and scientific inquiry, changing the landscape of research and paving the way for future discoveries.
The Path Ahead for Microscopy
Looking towards the future, microscopy holds immense promise. The field is poised for further innovations that can refine existing techniques while introducing entirely new approaches. Anticipatory developments may include enhanced computational capabilities for real-time analysis or integration with advanced imaging modalities.
Moreover, a multidisciplinary approach that combines biology, materials science, and artificial intelligence will likely lead to breakthroughs. The ability to efficiently process and analyze vast sets of data will unlock new dimensions of research previously thought unattainable.
The potential for collaboration among researchers from various specialties is invaluable. As microscopy continues to evolve, its role in addressing global challenges such as healthcare and environmental sustainability will become increasingly vital. The path ahead is not only about technological advancements but also about fostering the scientific spirit of discovery and inquiry.
"Microscopy is a gateway to understanding the intricacies of life and materials at a scale previously unreachable by human observation."