The Essential Role of Batteries in Electric Vehicles
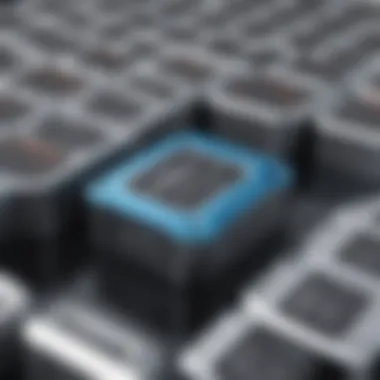
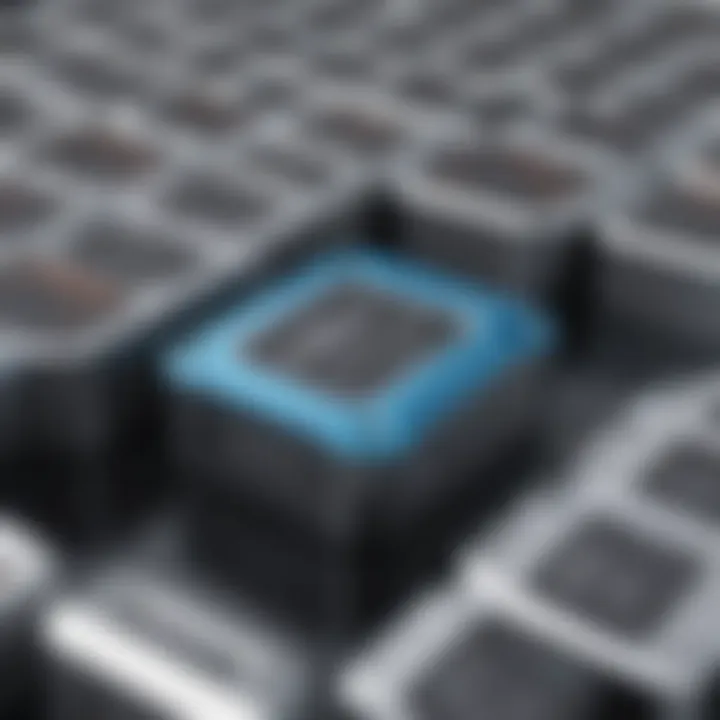
Intro
As the world pivots towards greener alternatives for transportation, electric vehicles (EVs) are capturing the spotlight. But at the heart of this revolution lies an element that often goes overlooked: the battery. The battery serves not simply as a power source but is intricately involved in defining the performance, efficiency, and longevity of electric vehicles. Understanding how these batteries function, their design, and their chemistry is crucial for anyone interested in the future of sustainable mobility.
In the following sections, we will explore the different battery technologies prevalent in the electric vehicle market today. Not only will we dive into lithium-ion batteries, which have been the workhorses of EVs, but we will also examine solid-state batteries and other emerging alternatives. Each type has its own strengths and weaknesses, affecting everything from energy density to safety.
Moreover, weโll discuss the relationship between battery efficacy and environmental considerations, as well as the economic implications tied to these technologies. Ultimately, understanding batteries means understanding the future trajectory of electric vehicles, which holds great promise for a sustainable transportation landscape.
This article aims to provide a deep dive for students, researchers, educators, and professionals. The goal is to bring clarity to a complex topic and highlight the advances that could steer us toward a more energy-efficient future.
The Importance of Batteries in Electric Vehicles
Batteries serve as the lifeblood of electric vehicles, playing an invaluable role in their functionality and efficiency. Without them, these modern marvels would be nothing more than a curious concept, driven by a tether to charging stations rather than the open road. As the automotive landscape shifts towards sustainability, understanding the importance of batteries becomes essential not only for manufacturers but also for consumers on the brink of this EV revolution.
Defining the Electric Vehicle Landscape
To appreciate the significance of batteries, one must first comprehend the electric vehicle landscape. Traditional vehicles, reliant on the combustion of fossil fuels, produce emissions that contribute to global warming and environmental degradation. In contrast, electric vehicles promise a cleaner future, harnessing electricity to power their motors.
Electric vehicles generally encompass different models, from hybrids that combine internal combustion engines with electric power to fully electric vehicles like the Tesla Model S or the Nissan Leaf. The breadth of options, however, hinges on one common denominator: the battery. Itโs the component that enables these vehicles to store and utilize energy efficiently, transforming electric power into kinetic motion. As the curtain lifts on this electric era, the global market is witnessing an incremental shiftโmore consumers are opting for cleaner emissions, thus increasing the demand for robust and reliable battery technology.
Role of Batteries in EV Functionality
Batteries in electric vehicles do more than just store energy; theyโre the key facilitators of the whole system. The vehicle's operational efficiency, driving range, charge times, and overall performance are significantly governed by battery technology. Here are some primary roles that batteries play in electric vehicles:
- Energy Storage: At their core, batteries are designed to store energy generated from the grid, solar panels, or regenerative braking systems.
- Power Delivery: During acceleration, the battery must deliver power quickly to propel the vehicle forward, necessitating efficient design precision to ensure performance.
- Range Management: Batteries influence how far an electric vehicle can travel on a single charge. Larger, high-density batteries can store more energy, which translates to increased driving range.
- Thermal Management: They must also maintain optimal operating temperatures. Battery performance can wane if they become too hot or too cold, thus necessitating systems to manage heat effectively.
"The battery serves as the heart of the electric vehicle, pumping energy that fuels the drive toward sustainability."
The evolution of battery technology directly impacts the electric vehicle's capabilities, pushing the boundaries of what is possible. As manufacturers invest in research and innovations, understanding how these batteries function is paramount. The intersection of battery technology and electric vehicles paves the way for advancements that could transform personal transportation into a more sustainable and efficient system.
In summary, the significance of batteries in electric vehicles cannot be overstated. They are not mere components; rather, they are the foundational elements that determine the efficiency, performance, and appeal of electric vehicles. A solid grasp of their role leads to better informed decisions, whether youโre a potential EV buyer or a stakeholder in the automotive industry.
Battery Technologies Overview
Batteries are at the heart of electric vehicles. Their technology deeply influences performance, efficiency, and sustainability. Understanding the landscape of battery technologies helps contextualize advancements that empower todayโs electric mobility. This section delves into the nuances of various battery types, highlighting their characteristics, benefits, and some vital considerations. By comprehending these elements, stakeholders can make informed decisions as the market evolves.
Lithium-Ion Batteries
Lithium-ion batteries are the reigning champions of the electric vehicle world, often praised for their balance of energy density and efficiency. To put it simply, they store more energy in a smaller footprint compared to older technologies. This high energy density is critical for electric cars since it allows for longer driving ranges on a single charge.
Moreover, lithium-ion batteries enjoy relatively low self-discharge rates, which means they can hold a charge longer when not in use. However, they aren't without their snags. Safety is a pressing concern; if damaged or improperly handled, these batteries can catch fire or even explode. Additionally, the manufacturing process has environmental implications, particularly concerning lithium extraction, which can be water-intensive and socially contentious.
"The choice of battery technology in electric vehicles directly impacts their usability, sustainability, and safety."
Solid-State Batteries
Solid-state batteries promise to revolutionize the electric vehicle landscape by replacing the liquid electrolytes seen in lithium-ion technology with solid materials. This shift could significantly enhance safety, as solid-state designs are less susceptible to leaks and thermal runaway conditions. Consequently, the risk of fire is dramatically reduced, making these batteries appealing for consumer confidence.
Additionally, solid-state batteries could potentially offer higher energy density than their liquid counterparts, translating to longer ranges for electric vehicles. However, this technology is not yet in widespread commercial use due to challenges in large-scale production and cost. Current research efforts are aimed at overcoming these barriers, making it an exciting area to watch within battery technology.
Emerging Battery Technologies
Beyond lithium-ion and solid-state options, a wave of emerging battery technologies is set to shake things upโnot only in electric vehicles but in the broader energy storage market. From lithium-sulfur to sodium-ion batteries, researchers are exploring various chemistries that could mitigate some of the limitations of existing technologies. For example, lithium-sulfur batteries promise a higher theoretical capacity than traditional lithium-ion types, which means they could store more energy effectively.
However, the road is not without potholes. Many emerging technologies involve trade-offs in durability, cost, or scalability. As they move from lab environments to real-world applications, their usability in larger volumes remains a topic of active research and development.
The dynamic field of battery technology is critical to advancing electric vehicles. The continued evolution and adoption of new battery technologies will play a pivotal role in the future of sustainable transportation.
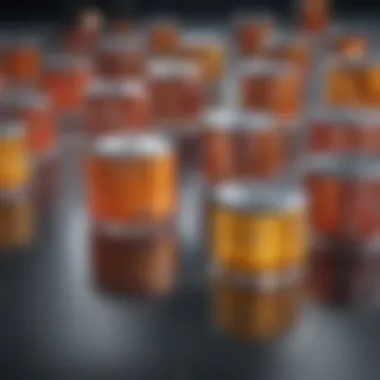
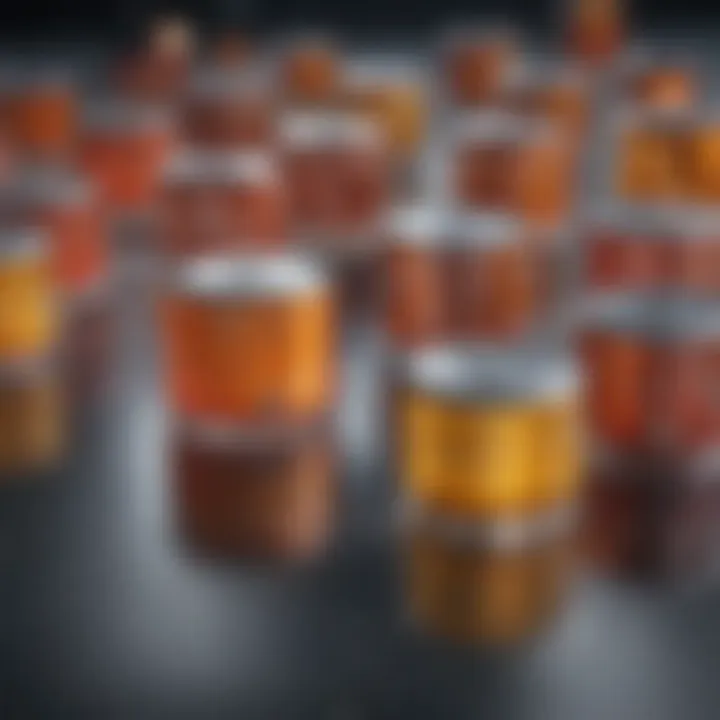
Battery Chemistry: Components and Reactions
Battery chemistry plays a pivotal role in the functionality and efficiency of electric vehicles. At its core, this branch dives into the materials and reactions that take place in batteries, shaping not just the power output but also the lifespan of these fundamental components. Understanding this chemistry helps to unravel the intricate dance between energy storage and release, providing insight into performance metrics that consumers and manufacturers alike find crucial.
In this vein, battery chemistry is not merely a technical subject; itโs intertwined with aspects of sustainability, cost efficiency, and the broader adoption of electric vehicles. As this field advances, consumer awareness about these chemical processes enhances trust and acceptance towards electric mobility, moving us closer to a greener automotive future.
Key Materials in Battery Construction
When discussing battery chemistry, itโs important to zero in on the key materials that form the building blocks of batteries. Most batteries fundamentally comprise electrodes, electrolytes, and separators, each with specific materials that impact their performance.
- Electrodes are typically made from lithium cobalt oxide for the cathode, offering high energy density, while the anode often uses graphite. This combination is pivotal for lithium-ion batteries.
- Electrolytes serve as the medium enabling ion transfer between electrodes. LiPF6 (lithium hexafluorophosphate) is common in lithium-ion batteries, providing conductivity while maintaining stability.
- Separators are essential for preventing direct contact between the electrodes. Generally made from materials such as polyethylene or polypropylene, they allow lithium ions to pass while keeping the electrodes apart to avoid short-circuiting.
In addition, itโs worth noting that ongoing research is keen on finding alternative materialsโlike silicon anodesโwhich could further increase energy density and efficiency. The pursuit of cleaner and more sustainable materials signals an enlightening shift towards greener technologies in battery production.
Electrode Reactions and Their Implications
The magic of battery operation lies in the electrode reactions, which capture the essence of energy conversion. During discharging, lithium ions flow from the anode to the cathode, undergoing oxidation in the anode and reduction in the cathode. This movement of ions not only generates electricity but also illustrates the inherent reversibility of these reactions.
The reactions are defined as follows:
- At the anode:
LiC6 โ Li+ + e- + C6
(Lithium ions are released into the electrolyte and electrons into the circuit.) - At the cathode:
LiCoO2 + Li+ + e- โ Li2CoO2
(Lithium ions slot into the cathode material, storing energy.)
Such chemical transformations are not without their consequences. Factors like the rate of charge and discharge can significantly affect the longevity and health of a battery. The efficiency of these reactions can lead to heat generation, which, if unmanaged, can exacerbate degradation. Understanding these reactions is vital for advancing battery technology.
"The journey, not the destination, matters when aiming for efficiency in battery technology"
In essence, being aware of how key materials interact during operation paints a clearer picture of performance and reliability in electric vehicles. As innovations surface, the hope is to harness this chemistry more effectively, ultimately propelling us toward a future where batteries are not just energy storage devices, but central figures in the quest for sustainable transportation.
Performance Metrics of EV Batteries
In the realm of electric vehicles (EVs), performance metrics of batteries are paramount for assessing not only the efficiency of the vehicle but also its viability as a sustainable option in transportation. These metrics encompass factors such as energy density, charging speed, and battery lifespan, each playing a critical role in shaping the user experience and the overall effectiveness of EVs.
Understanding these performance metrics allows engineers, manufacturers, and consumers to benchmark different batteries and make informed decisions concerning technology investments and vehicle choices. Moreover, as the demand for electric vehicles continues to rise, performance metrics become more significant in aligning battery capabilities with user expectations in terms of range and reliability.
Energy Density and Its Importance
Energy density is a term tossed around often in discussions of batteries, yet its significance cannot be overstated. It refers to the amount of energy stored per unit of weight or volume, typically measured in watt-hours per kilogram (Wh/kg) for weight and watt-hours per liter (Wh/l) for volume. The higher the energy density, the more energy batteries can hold, ultimately impacting how far an EV can travel on a single charge.
This characteristic is particularly vital in urban and highway driving scenarios. For instance, a vehicle equipped with a battery that showcases high energy density can offer longer ranges, making it a more attractive choice for consumers who want to avoid frequent charging stops. Furthermore, as manufacturers strive to create lighter and more efficient vehicles, enhanced energy density translates to lighter battery packs, which could lead to improved vehicle performance.
"Energy density directly impacts range; a higher density means further journey without recharging."
Charging Speed and Infrastructure Considerations
Charging speed, a key performance metric, signifies how quickly an EV's battery can recharge. This isn't just a matter of convenience; it's foundational to the widespread adoption of electric vehicles. As prospective buyers consider transitioning from gasoline-powered vehicles to electric ones, the availability and effectiveness of charging infrastructure weigh heavily on their decision.
Fast-charging technologies have been gaining traction, with some batteries now capable of achieving an 80% charge in under 30 minutes. This lightning-fast capability could significantly reduce what is often viewed as a major drawback of EVsโcharging time. However, it's not just about the battery; robust and extensive charging networks are equally essential.
To navigate this, various stakeholders from governments to private companies are investing in developing fast-charging stations. Cities like Amsterdam and San Francisco are paving the way with their charging infrastructure, showing how effective policies can shift consumer attitudes towards EV adoption.
Battery Lifespan and Degradation Factors
Battery lifespan is the third pillar of battery performance metrics, often determined by how well a battery maintains its capacity over time. Generally lasting between 8 to 15 years in EVs, the actual life can vary based on multiple factors, including charge cycles, temperature, and usage patterns. One major concern is degradation, the gradual loss of a battery's ability to hold energy as it undergoes numerous charge and discharge cycles.
Knowingly caring for batteries can extend their life. For example, keeping batteries between 20% and 80% charge can help prevent unnecessary stress that leads to degradation. Additionally, temperature extremes can dramatically affect battery life; cold temperatures can reduce performance, while heat can accelerate wear and tear.
Understanding these elements is crucial for both manufacturers and consumers. Educated decisions regarding charging habits and maintenance can lead directly to better performance outcomes and cost savings in the long run.
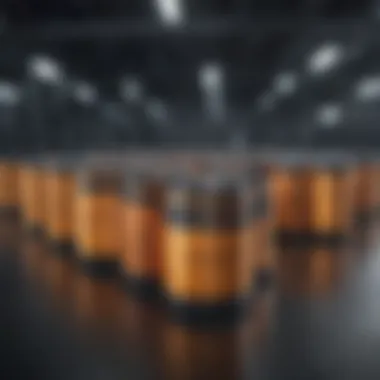
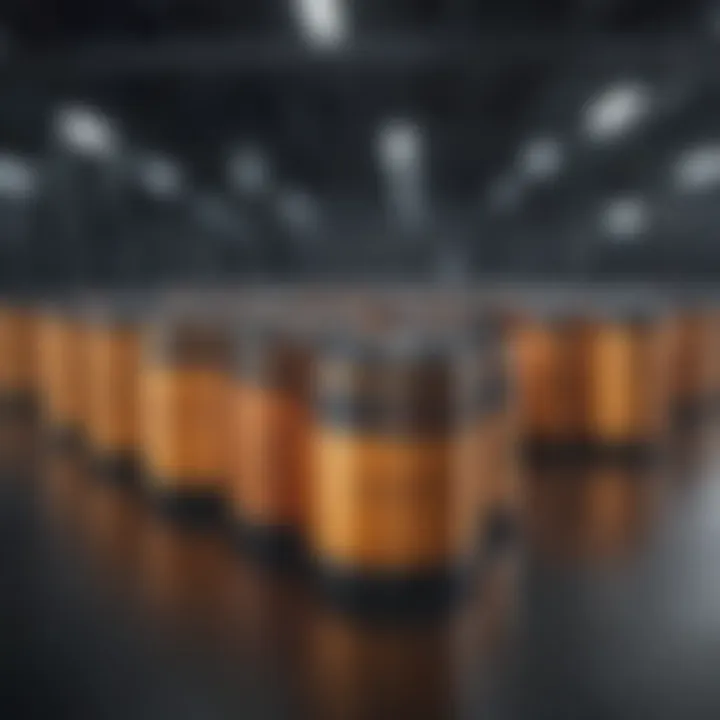
Environmental Considerations
Batteries, the lifeblood of electric vehicles, donโt just affect how well these cars perform; they also carry significant environmental footprints. Addressing environmental considerations in battery production and disposal is essential for a sustainable future. Itโs not just about whatโs under the hood; itโs about the whole lifecycle of the battery system that the world needs to grapple with.
Impact of Battery Production on Resources
The production of batteries, especially lithium-ion ones, raises eyebrows when it comes to the environment. It involves extracting raw materials like lithium, cobalt, and nickel from the earth, and this process can be quite drainful for local ecosystems. For instance, lithium extraction in places like the Chilean salt flats is not just a matter of digging stuff out of the ground; it uses vast amounts of water, affecting local communities and wildlife. If you think about it, the environmental costs can outweigh some of the benefits that EVs bring later on.
Furthermore, there's much talk about cobalt mining, especially in the Democratic Republic of the Congo. Much of the mining is done by hand, often under unsafe conditions, and the waste left behind can leach harmful substances into the soil and waterways. It's a double-edged swordโthe faster the world moves toward electric vehicles for climate reasons, the more it risks worsening some environmental and social issues related to battery production.
This creates a pressing need for innovations in sourcing materials, such as developing methods for recycling materials already in circulation. We canโt just keep digging; there needs to be a balance between demand and responsible sourcing. With demand for electric cars set to soar, the pressure is on to ensure that production doesnโt exploit resources or create new environmental hazards.
Recycling and Circular Economy in Battery Production
Recycling may not be the first word that comes to mind when discussing batteries, but itโs a vital part of the conversation. The idea of a circular economy essentially flips the traditional model on its head. Instead of chasing down new materials at the cost of the planet, the focus shifts to reusing and recycling existing batteries. Why let those materials go to waste when they can power the next fleet of electric vehicles?
Currently, battery recycling is like a well-kept secret that not many seem to know about. Yet, it's got enormous potential. Unused or end-of-life batteries can be efficiently repurposed within new products. For example, car manufacturers can recover up to 95% of the materials in lithium-ion batteries, which is a huge step towards minimizing waste.
Recycling not only reduces the ecological impact of mining new materials but also helps mitigate supply chain vulnerabilities. With materials like lithium becoming rarer due to high demand, being able to recover and reuse helps keep production costs in check. It simply makes sense to leverage whatโs already out there.
Here are a few critical components of a robust recycling system:
- Collection Programs: Efficient collection schemes allow consumers to return spent batteries easily.
- Advanced Technologies: Innovations in recycling technology can improve recovery rates.
- Regulatory Support: Governments can encourage recycling through policies that promote sustainable practices.
In sum, focusing on recycling and a circular economy could serve as a driving force in maintaining environmental responsibility within the electric vehicle battery sector. Itโs about ensuring that the green technology we aim for doesnโt just create a new set of problems while fixing the old ones. The road ahead is clear: we canโt just think about making electric vehicles; weโve got to think about what happens after.
"As we move forward, the conversation about EV batteries cannot shy away from their environmental impactsโboth in construction and disposal."
Market Trends and Future Directions
The landscape of electric vehicles (EVs) is changing rapidly, and understanding the market trends and future directions of battery technology is vital for industry stakeholders and consumers alike. The increasing demand for sustainable transportation options has prompted a surge in innovation, making it paramount to examine how these trends will shape battery development moving forward. This section will delve into two specific facets: the impact of rising demand for EVs and the substantial investments driving technological advancements in batteries.
Demand for EVs and Its Influence on Battery Development
The interest in electric vehicles has exploded over the last few years. "Going green" is no longer just a fad; itโs become a lifestyle for many, and people are especially eager to explore the benefits of EVs. Thereโs a palpable shift from traditional combustion engines toward electric options. This mounting demand has a direct correlation with battery development.
As sales of electric vehicles continue to rise globally, manufacturers face the pressing challenge of producing batteries that meet heightened performance standards. Potential buyers are looking for vehicles that not only have a decent range but can also charge quickly and last long. This has put pressure on battery producers to invest in research and development that leads to both enhanced efficiency and affordability.
Some factors to consider include:
- Range Anxiety: People often fear that their EVs won't have enough battery life for longer trips. This promotes the development of higher-capacity batteries.
- Green Image and Regulations: With stricter regulations on emissions, car manufacturers are incentivized to produce more energy-efficient and greener battery technologies.
- Consumer Expectations: As technology evolves, consumers expect more from their vehiclesโthis includes faster charging times and greater longevity.
In short, the demand for electric vehicles directly incentivizes advancements in battery technology, which in turn influences consumer choices, market dynamics, and even regulatory policies.
Investment in Battery Technology Innovations
Another pivotal aspect of the future of battery technologies is investment. Thereโs a concerted effort from governments, private sectors, and venture capitalists to funnel money into battery research and innovation. This funding isnโt just trickling in; it's pouring.
Investments are focusing on various key areas:
- Research and Development: New battery chemistries, like solid-state batteries, are garnering attention due to their potential to significantly outperform conventional lithium-ion batteries.
- Manufacturing Processes: Money is being funneled into more efficient manufacturing processes, which can scale with the rising demand for batteries in EVs.
- Recycling and Sustainability: Even the end-of-life management of batteries is getting its due attention. Innovations in recycling techniques can contribute to a more sustainable lifecycle of battery materials.
The overarching goal of these investments is to realize a future where battery technologies can support not just electric vehicles but also larger applications like renewable energy storage systems.
"As we look toward a more electrified world, the role of innovative battery technologies cannot be overstated. They are critical to driving the success of electric vehicles and, indeed, the sustainability of our planet."
Government Regulations and Policies
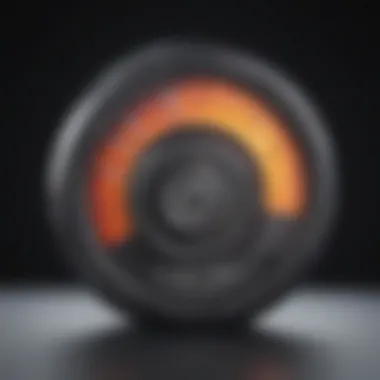
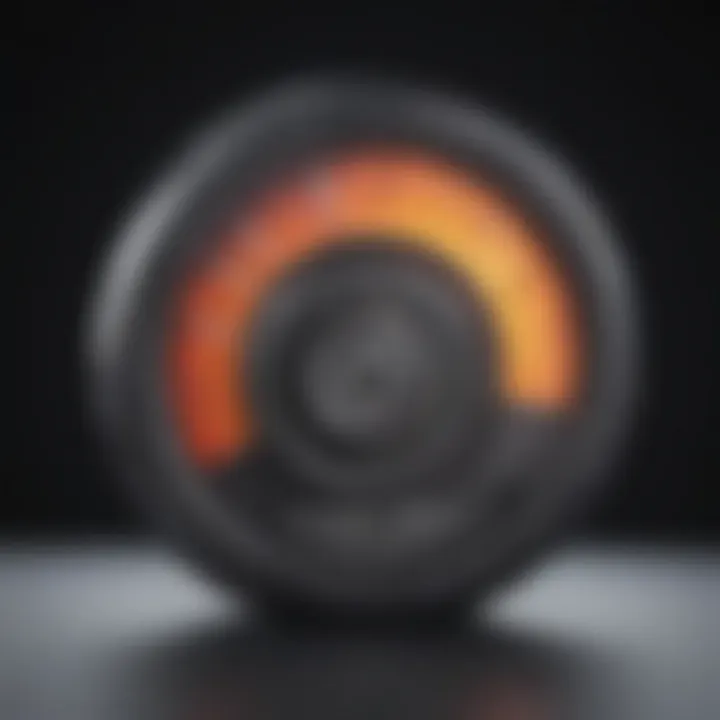
Government regulations and policies play a pivotal role in shaping the landscape of battery technology and, by extension, the electric vehicle industry. As the world pivots towards sustainable energy, understanding these regulations is vital for driving effective changes in battery development, usage, and recycling practices. Policies can directly influence market dynamics, affecting how quickly innovations emerge and how well existing technologies are implemented. In this section, we'll explore the motivations behind the regulations, the incentives for research and development, and the necessary frameworks for responsible manufacturing and disposal.
Incentives for Battery Research and Development
Governments across the globe recognize the critical importance of advancing battery technologies. This recognition translates to the provision of incentives aimed at accelerating research and development in the field. Incentives can take many forms, including grants, tax breaks, and favorable loans for companies working on innovative battery solutions.
- Financial Support: For instance, countries like Germany have implemented substantial subsidies for battery research initiatives. These funds can help startups and established companies alike to explore cutting-edge battery technologies that might not receive private investment due to perceived risks.
- Collaborative Programs: Many nations also promote collaborative programs between universities and industry experts. Such partnerships can foster innovative approaches that advance battery efficiency and sustainability.
- Public-Private Partnerships: Dedicated entities, like the Advanced Research Projects Agency-Energy in the United States, work hand-in-hand with private businesses. This collaboration not only nurtures innovation but also aligns projects with governmental sustainability goals.
Ultimately, these incentives aim to lower the risks associated with pioneering new technologies. By reducing barriers for research funding and supporting knowledge sharing, governments can fast-track advancements that directly impact electric vehicle performance, reliability, and environmental friendliness.
Regulations on Battery Manufacturing and Disposal
The push for cleaner energy solutions doesn't merely focus on production but also emphasizes responsible disposal and recycling. Regulations surrounding battery manufacturing and disposal are essential to ensure that the entire lifecycle of batteries aligns with sustainability principles.
- Manufacturing Standards: Governments are instituting stringent manufacturing standards that dictate how batteries are produced, focusing on minimizing pollutants and encouraging the use of sustainable materials. For example, the European Union has established frameworks to ensure that battery production meets environmental guidelines, pushing manufacturers to use more recyclable materials.
- Disposal Protocols: Furthermore, there are strict protocols in place for battery disposal. Since batteries often contain hazardous materials, proper disposal is crucial for environmental protection. Regulations require manufacturers to establish take-back programs, ensuring that used batteries are returned and processed safely, thus reducing the negative impact on landfills and groundwater.
- Recycling Mandates: These regulations also advocate for the recycling of battery components. By mandating a percentage of recycled materials in new batteries, governments encourage the circular economy, which is the aim to minimize waste and resource consumption.
Helping to reduce pollution and improve energy efficiency, these measures foster a sustainable path forward for the battery industry and electric vehicles as a whole.
In summary, understanding government regulations and policies offers insights into how legislative frameworks support battery innovation and sustainability. With ongoing investments in research and clearer regulatory pathways, the future of battery technology in electric vehicles looks promising and is aimed at creating a cleaner world.
Consumer Awareness and Education
In the growing landscape of electric vehicles (EVs), consumer awareness and education play a pivotal role in driving advancements and adoption of battery technologies. Having a well-informed public is essential not only for making educated choices about personal transportation but also for contributing to a more sustainable future. Understanding how batteries work, their specifications, and the upkeep they require can empower consumers, making them more discerning buyers and users of EV technology.
Understanding Battery Specifications
Electric vehicle batteries come in various types, sizes, and designs. It's crucial for consumers to grasp the specifications that matter most. Key specifications include:
- Capacity (kWh): This determines how much energy the battery can store, directly affecting the range of the vehicle. A typical EV battery might range from 30 kWh to over 100 kWh.
- Voltage (V): Generally relates to the power output of the battery. Higher voltage systems can deliver more power, crucial for performance.
- Charge Cycles: This indicates how many times the battery can be charged before its capacity diminishes significantly. Expected cycle life can range from 500 to 1,500 cycles depending on the technology.
- Energy Density (Wh/kg): This defines how much energy is stored in a given mass, influencing vehicle weight and range.
These specifications are akin to the fine print in a contract; they may seem less interesting at first glance but are otherwisely vital. In understanding these terms, consumers gain insight into how their choice of battery impacts the EV's overall performance and longevity. Furthermore, being aware of battery chemistry helps buyers discern between options like lithium-ion versus solid-state batteries, leading to more thoughtful decisions.
DIY Maintenance and Care for EV Batteries
Maintaining an EV battery might sound a bit daunting, but it boils down to a few straightforward practices that can enhance lifespan and efficiency. Here are some essential considerations for DIY maintenance:
- Regularly Monitor Charge Levels: Keeping the battery within optimal charge range is pivotal. Over-discharging can lead to lasting damage. A sweet spot is often between 20% and 80% charge.
- Optimal Charging Tempo: Fast charging is convenient but can generate excess heat leading to degradation. Whenever possible, use standard charging methods to protect battery life.
- Battery Temperature Management: Extreme heat or cold can affect performance and damage battery cells. If possible, park in shaded or temperature-controlled areas to regulate battery temperature better.
- Check for Software Updates: Many EVs come equipped with systems that optimize battery performance through software. Keeping these up-to-date ensures the best functioning through continuous improvements.
By grasping these insights, consumers can take a proactive stance in their EV battery management. Utilizing recommendations and stretching the lifespan of their batteries not only provides economic benefits but contributes to a healthier environment through sustainability.
"An educated consumer is the best consumer; knowledge about battery specifications and maintenance transforms users into advocates for sustainable technologies."
In summary, fostering awareness and promoting education regarding the intricacies of EV batteries is not merely a matter of convenience but a vital step towards a future where electric vehicles can thrive. Empowered consumers can demand better products, support innovation, and ultimately contribute to greener, more sustainable practices in transportation.
The Future of Batteries in EVs
The trajectory of batteries in electric vehicles is poised to redefine our transportation landscape. As the push for sustainability intensifies, the innovation and evolution in battery technology become a focal point for researchers, manufacturers, and, ultimately, consumers. Understanding how these changes will unfold is crucial not just for industry insiders but also for anyone interested in the future of green technology and electric mobility.
Predictions for Battery Technology Developments
In the coming years, the battery sector is expected to undergo significant transformations that will have far-reaching implications. These include not only advancements in energy density but also improvements in safety and efficiency.
- Enhanced Energy Density: Future batteries may achieve much higher energy densities, allowing vehicles to travel longer distances on a single charge. This advancement could pave the way for electric vehicles to rival traditional combustion engine cars in terms of range.
- Reduced Charging Times: As fast-charging technologies evolve, the time it takes to recharge EV batteries may be drastically shortened, potentially making electric vehicles as convenient as refueling gasoline cars.
- Revolutionary Chemistry: The exploration of alternatives like lithium-sulfur and solid-state batteries could become the new norm. Such batteries promise better performance metrics and less reliance on scarce materials, making EVs more sustainable.
"The future of batteries is not solely about increasing capacity; itโs about creating an ecosystem of technology that supports sustainable and efficient transportation.โ
Innovations on the Horizon: Looking Ahead
Looking towards the horizon, one canโt help but be intrigued by the array of innovations just waiting to burst onto the scene. From manufacturing processes to real-world applications, several advancements are on the verge of making waves.
- Smart Batteries: The advent of smart battery technology means improved monitoring and management, optimizing performance and prolonging lifespan. These batteries would use AI algorithms to analyze usage patterns and environmental conditions, enhancing user experience.
- Wireless Charging: Imagine just driving your EV onto a specially designed surface that charges your vehicle without the fuss of plugging in. This technology is already under trial, and if successful, could change the way we think about recharging entirely.
- Battery-as-a-Service Models: As the demand for EVs increases, so does the variability in consumer needs. Battery leasing might emerge as a common model, making EV ownership more accessible while enabling continuous upgrades on battery performance without requiring outright purchases.
In summary, the evolution of batteries in electric vehicles isnโt merely a technological upgrade; it stands as a monumental shift in the very essence of transportation. As these trends continue to evolve, they carry with them the potential to reshape user experience, environmental sustainability, and the automobile industry as a whole.