The Role of the Anode in Electrolysis Explained
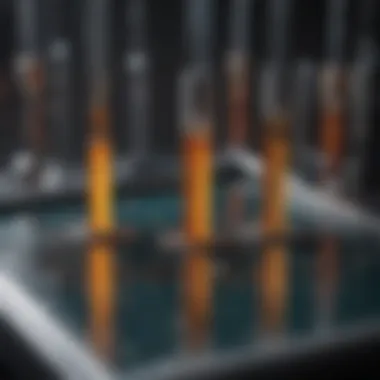
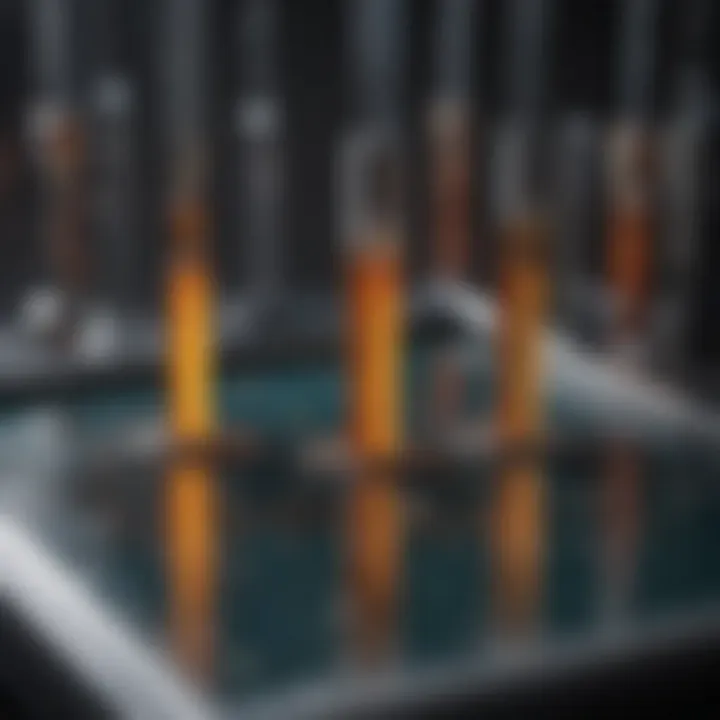
Intro
Electrolysis represents a pivotal process with expansive applications, notably in metal extraction and hydrogen production. At the heart of this process lies the anode, which serves as the site for oxidation reactions. Understanding the anodeβs role is critical for scholars and professionals engaged in various scientific and industrial fields. Not only does it affect the efficiency of the electrolysis process, but advancements in anode technology are also shaping future research and applications.
By comprehensively analyzing the significance of the anode, this article aims to elucidate its materials, functions, and the electrochemical mechanisms at play. Through this examination, we can better appreciate how the anode influences the efficiency of electrolysis and the broader implications for industry and research.
Research Overview
This section presents a summary of key findings and outlines the objectives that frame the current investigation into the role of the anode in electrolysis.
Summary of Key Findings
- The anode plays a vital role in determining the efficiency of electrochemical reactions.
- Different materials, such as titanium and platinum, demonstrate varying performance that influence reaction rates.
- The choice of anode material can significantly affect the overall cost and environmental impact of electrolysis processes.
Research Objectives and Hypotheses
The primary objective of this research is to analyze the influence of anode materials and design on the efficiency of electrolysis. Specific hypotheses include:
- The utilization of advanced materials will improve the efficiency of oxidation reactions occurring at the anode.
- Innovations in anode technology will lead to reduced energy consumption in electrolysis.
Methodology
Here, we outline the study design and approach taken in this research to better understand the role of the anode.
Study Design and Approach
This research employs a comparative analysis of various anode materials through experimental electrolysis setups. By measuring current production and reaction efficiency, we are able to draw conclusions about each material's effectiveness.
Data Collection Techniques
Data will be collected through:
- Electrochemical Testing: Measurements of voltage, current, and efficiency will be systematically recorded during experiments.
- Material Analysis: After testing, anode materials will be analyzed to assess surface changes and degradation.
By establishing this framework, the research seeks to contribute valuable insights into how anode function and material choice will affect future developments in electrolysis.
Intro to Electrolysis
Electrolysis is a significant process that facilitates the decomposition of compounds into their elemental constituents through the application of electrical energy. The importance of this topic lies in its widespread applications across various fields, including metal extraction, chemical synthesis, and hydrogen production. Understanding the fundamentals of electrolysis sets the stage for an in-depth exploration of its components, particularly the anode. The anode, as one of the critical electrodes, plays a pivotal role in facilitating oxidation reactions that are at the heart of the electrolysis process.
Definition and Principles of Electrolysis
Electrolysis involves passing an electric current through a conductive solution, often termed an electrolyte, causing chemical reactions at the electrodes. The fundamental principle revolves around the transfer of electrons. When the current flows, cations migrate towards the cathode, where reduction occurs, while anions move towards the anode, where oxidation takes place. This movement of charged particles is crucial in both industrial and research applications. The process can be summarized by the equation:
- Cations gain electrons (reduction) at the cathode.
- Anions lose electrons (oxidation) at the anode.
This dual action is driven by the electric potential and the nature of the electrolyte.
Historical Context of Electrolysis
The concept of electrolysis has evolved significantly since its inception in the early 19th century. Sir Humphry Davy, Michael Faraday, and other prominent scientists laid the groundwork for our current understanding of electrolysis. Faradayβs laws of electrolysis, formulated in 1832, provide the foundational framework that quantifies the relationship between the amount of substance transformed at the electrodes and the quantity of electricity used. Historical developments showcase the transition from theoretical knowledge to practical applications, emphasizing the role of electrolysis in various industrial processes such as electroplating, electrorefining, and the production of chlorine and sodium hydroxide.
"Electrolysis not only advances industrial capabilities but also bridges the gap between fundamental chemistry and practical applications."
This historical background illustrates the necessity of comprehending electrolysis to achieve proficiency in the field.
Understanding the Anode
The anode plays an essential role in the electrolysis process. It is the site where critical oxidation reactions occur, making it indispensable for various applications like metal extraction and hydrogen production. Understanding the anode allows researchers and professionals to optimize its performance and improve the efficiency of electrolysis systems.
Within electrolysis, the anode has unique functions that contribute to overall chemical transformations. The material composition, shape, and surface characteristics of the anode can significantly affect its performance and longevity. By exploring these elements, we can identify the best practices for anode design and usage, ensuring maximum efficacy in chemical reactions.
Definition and Role of the Anode
The anode is defined as the electrode where oxidation occurs during electrolysis. This involves the loss of electrons from the substances in the vicinity of the anode. In simple terms, it serves as the positive electrode in an electrochemical cell. Upon an external electrical current being supplied, positive ions move toward the anode, where they undergo oxidation. This fundamental process is crucial for the functioning of various electrolytic systems.
Furthermore, the type of reactions happening at the anode affects the overall efficiency of electrolysis. Different materials possess distinct electrical and chemical properties that determine how well they catalyze oxidation reactions. More conductive materials can facilitate quicker reactions, while those resistant to corrosion can endure longer operational periods.
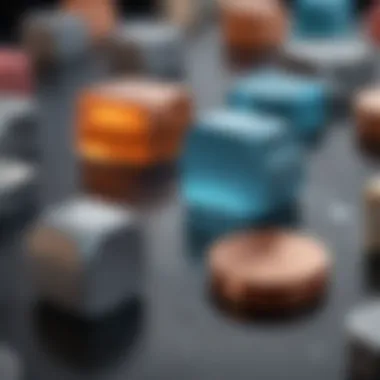
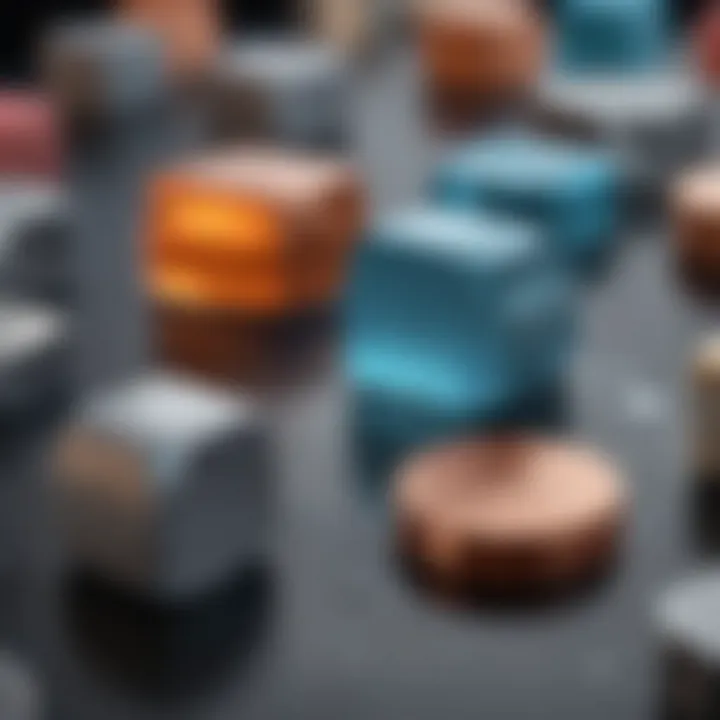
Oxidation Reactions at the Anode
Oxidation reactions at the anode are vital for driving the overall electrolysis process. At the molecular level, these reactions involve the release of electrons, which can then be captured by other substances in the electrolyte. This transfer of electrons is what enables the electrochemical reaction to continue.
To illustrate, in water electrolysis, oxygen molecules are formed at the anode through the oxidation of water. The half-reaction can be summarized as:
[ 2H_2O(l) \rightarrow O_2(g) + 4H^+(aq) + 4e^- ]
This equation highlights both the physical and chemical changes that occur during oxidation. Understanding these reactions allows for better prediction and control of reaction dynamics, significantly impacting practical applications.
The success of electrolysis heavily relies on the efficiency of anode reactions.
In different contexts, such as metal recovery, various metals may oxidize differently at the anode, demanding specific considerations based on the processes in use. Studying these oxidation reactions not only improves existing applications but also opens paths for innovation in future electrochemical technologies.
Materials Used for Anodes
The selection of materials for anodes is a critical consideration in the field of electrolysis. The efficacy of an electrolysis system depends significantly on the materials used for the anode. Proper material choice not only influences the efficiency of oxidation reactions but also enhances the durability and lifespan of the anode itself. Additionally, the characteristics of various materials can impact their performance under operational conditions, making this a vital area of exploration.
Common Anode Materials
In electrolysis, several materials are commonly used for anodes due to their favorable electrochemical properties. Some of the principal materials include:
- Graphite: This material is well-known for its excellent conductivity and resistance to corrosion. Graphite anodes are widely used in electrolytic processes, especially in metal plating and purification applications.
- Platinum: Although costly, platinum serves as a highly effective anode material because of its remarkable chemical stability and conductivity. Its use is prominent in water splitting and other high-efficiency applications.
- Lead Dioxide: This material is flat out common in electrolysis cells, especially for producing chlorine and caustic soda. Its stability and reliable performance make it a standard choice despite concerns regarding lead toxicity.
These materials have been extensively researched and applied, proving their significance in practical electrolysis systems. Their unique properties allow for optimized electrochemical reactions, which enhance overall system performance.
Innovative Anode Materials
The landscape of anode materials is evolving, as research continues to focus on innovative solutions that promise to overcome the limitations of traditional materials. Some notable advancements include:
- Mixed Metal Oxides (MMOs): These materials combine various metal oxides to improve stability, conductivity, and efficiency. MMOs are becoming increasingly popular for their superior performance in various electrolysis applications.
- Carbon-based Nanomaterials: The use of graphene and other carbon nanostructures has shown potential in improving electrode performance. These materials can enhance conductivity and provide a large surface area for reactions, thus increasing efficiency.
- Conductive Polymers: This emerging category of materials offers flexibility and ease of processing. Conductive polymers may not always match the performance of conventional materials yet they provide options for creating more versatile systems.
Innovative materials can lead to significant improvements in electrolysis efficiency and broaden the range of applications. Their development continues to be a focal point in ongoing research, as they hold promise for sustainable and cost-effective solutions in the field of electrolysis.
The choice of anode material is pivotal not only for the efficiency of the electrolysis process but also for its economic viability.
In summary, understanding the range of materials available and their characteristics is crucial for optimizing anode performance in electrolysis. Common materials have established reliability, whereas innovative materials offer new opportunities for advancement.
Anode Design Considerations
In electrolysis, the design of the anode plays a pivotal role. Anode design considerations can significantly influence efficiency and performance during the electrochemical reactions. An effective anode must account for a range of factors including geometry, surface area, and conductivity. These elements are critical, as they directly impact the rate of oxidation reactions and the overall effectiveness of the electrolysis process.
The choice of anode material is equally important. It must not only support the desired electrochemical reaction but also withstand the harsh conditions present during operation. Properly designed anodes lead to better electron transfer, reducing energy consumption, and improving yield.
Geometric Configurations
The geometric configuration of an anode affects the uniformity of current distribution across its surface. A well-designed geometric structure can maximize surface area available for reaction while minimizing resistance to ion flow. Common shapes include flat plates, rods, and tubular structures. Each configuration has its benefits:
- Flat plates allow for even current distribution but may have limitations in active surface area.
- Rods generally provide higher surface area but can create uneven current densities.
- Tubular anodes often combine the advantages of both by providing more surface area while maintaining good flow dynamics.
Additionally, the spacing between the anode and cathode significantly affects performance. If the electrodes are too close, it may lead to increased resistance, while too much distance can result in inefficient ion transport. The geometry can thus be tailored to optimize the electrochemical performance of the cell.
Surface Area and Conductivity
Surface area and conductivity are intertwined factors in anode efficacy. The larger the surface area of an anode, the greater the number of reaction sites available, leading to increased reaction rates. Enhanced surface area can be achieved through various means, including the use of porous materials or coatings that increase texture or structural complexity.
Conductivity ensures that electrons are efficiently transported away from the anode during the oxidation reactions. High conductivity materials, such as platinum or certain graphitic structures, are often employed to ensure minimal energy loss during electron flow.
Factors such as surface smoothness, roughness, and the presence of active sites can enhance conductivity and efficiency in electrolysis processes.
Moreover, the choice of material impacts both surface area and conductivity. Innovative materials, like those incorporated with nanostructures, provide not just increased surface area but also promote efficient electron transfer.
In summary, considering geometric configurations and maximizing both surface area and conductivity are essential components of effective anode design. Identifying and implementing these factors in anode development can lead to significant improvements in electrolysis efficiency.
Electrochemical Mechanisms
Electrochemical mechanisms are crucial to understanding how electrolysis operates, especially regarding the role of the anode. In electrolysis, the anode is where oxidation occurs, and this process leads to the transfer of electrons and atoms. Understanding the underlying electrochemical mechanism helps in enhancing the efficiency of the electrolysis process and determining the optimal materials and conditions to be used in industrial applications.
Mechanisms of Oxidation Reactions
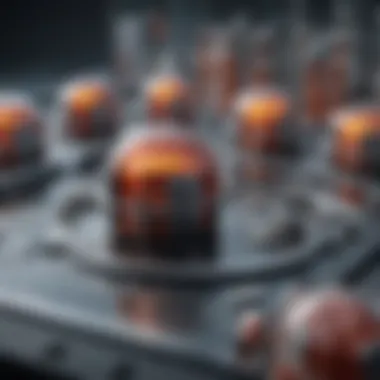
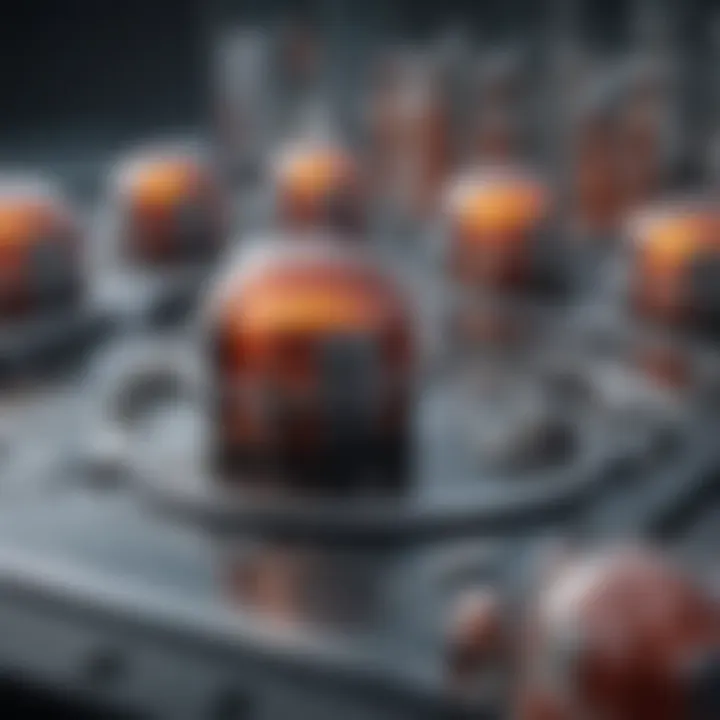
Oxidation reactions at the anode are the key to electrolysis. These reactions involve the loss of electrons from a substance. For example, in the electrolysis of water, oxygen atoms lose electrons at the anode, resulting in the formation of molecular oxygen. This process can be summarized in the following steps:
- Electron Loss: At the anode, the anions migrate towards the positive electrode, where they lose electrons. This loss marks the oxidation reaction, which is fundamental to driving the electrolysis process.
- Gas Evolution: The electrons lost by the anions often result in gas evolution. For instance, in water electrolysis, oxygen gas is released at the anode, which is crucial for applications such as hydrogen production.
- Formation of New Products: Depending on the electrolyte used, different products can emerge from oxidation reactions at the anode. This selectivity can be utilized to produce specific chemicals through electrolysis.
The efficiency of these oxidation reactions is influenced by the material composition of the anode, its surface area, and the overall conditions of the electrolysis process. Therefore, understanding these mechanisms is essential for optimizing reactions and improving overall yield.
Role of Electrons in Anodic Processes
Electrons play a fundamental role in anodic processes during electrolysis. When oxidation occurs, electrons are removed from the anode material and flow through an external circuit, typically into the cathode. This flow of electrons is what allows for the electrical current that drives the electrolysis reaction.
- Current Flow: The flow of electrons can be described by Faraday's laws of electrolysis, which relate the amount of substance altered at an electrode to the quantity of electricity passed through the system. The relationship underscores the importance of electron transfer efficiency in electrochemical reactions.
- Electron Dynamics: The dynamics of electron movements can affect the kinetics of the oxidation reactions. High electron mobility within the anode materials can enhance the reaction rates, leading to higher overall efficiency. This is of particular interest in research focused on improving anode materials.
- Effect of Anode Material: Different anode materials demonstrate various levels of conductivity and electron transfer capabilities. For instance, platinum, titanium, and graphite are commonly explored materials due to their favorable electrochemical properties.
In summary, the mechanisms of oxidation reactions and the role of electrons are key components in the efficiency of electrolysis. By studying these aspects, researchers can innovate and improve electrolysis technologies, leading to more effective applications in energy generation and chemical synthesis.
Understanding these mechanisms is essential for developing new approaches and methodologies to optimize electrochemical reactions. As electrolysis continues to gain importance within various industries, mastering these processes will prove beneficial for enhancing production efficiency and material utilization.
Electrolysis Efficiency and the Anode
Electrolysis is a process that relies heavily on the efficiency of its components, particularly the anode. The anode is pivotal for conducting oxidation reactions that underpin the entire electrolysis process. An efficient anode not only enhances the rate of reaction but also affects the energy consumption of the entire system. When discussing electrolysis efficiency, several factors tie back to the anode's performance. These inclusions underscore the relevance of the anode in applications ranging from metal extraction to environmentally sustainable hydrogen production.
Factors Influencing Anode Performance
Anode performance is influenced by multiple parameters. The main factors include:
- Material Properties: The intrinsic properties of the anode material, such as conductivity, thermal stability, and corrosion resistance, play significant roles. Materials like graphite and platinum are commonly used due to their favorable characteristics.
- Electrode Surface Area: A larger surface area allows for increased reaction sites, enhancing overall efficiency. Techniques such as porous designs or coating materials are often employed to achieve this.
- Current Density: The amount of current applied influences the rate of oxidation reactions. Operating at optimal current density is crucial for maximizing efficiency without causing unwanted side effects like passivation.
- Temperature and pH Levels: These environmental factors can significantly influence reaction kinetics at the anode. Adjusting temperature and pH according to specific needs can improve overall process efficiency.
- Electrolyte Composition: The type and concentration of ions in the electrolyte affect the anode's effectiveness. Selecting an appropriate electrolyte can optimize performance and thus electrolysis efficiency.
Impact of Anode Composition on Efficiency
The composition of the anode material has direct implications on its effectiveness. Various materials exhibit different electrochemical behaviors. For example:
- Noble Metals: Platinum and iridium are efficient, but their cost is a significant drawback. They have high tolerance to corrosion and provide excellent catalytic activity.
- Non-Noble Metals: Materials like nickel and copper are less expensive but might not offer the same level of performance.
- Composite Materials: Developing composite materials that combine various elements can tap into the benefits of multiple compositions. These composites can maximize efficiency while minimizing costs.
"Choosing the right anode material is fundamental to optimizing electrolysis efficiency, impacting both output and sustainability."
In summary, the efficiency of electrolysis processes is closely tied to the anode's performance. From the material properties to environmental conditions, all factors contribute significantly to efficiency. Understanding these elements is essential for enhancing the applications of electrolysis in industry and research alike.
Applications of Anodes in Electrolysis
The application of anodes in electrolysis stands as a pivotal component in various industrial processes. The significance of understanding these applications cannot be overstated, as they offer crucial insights into how electrolysis affects the modern world. Electrode material selection, oxidation reactions, and system design all play essential roles in determining the effectiveness and efficiency of processes, from metal extraction to renewable energy production.
Metal Extraction Processes
In the realm of metallurgy, anodes are integral to metal extraction methods, especially for non-ferrous metals like aluminum and copper. During electrolysis, metal ions are reduced at the cathode, while oxidation occurs at the anode. This not only helps to separate the desired metal from its ore but also assists in refining impurities. The choice of anode material, such as graphite or titanium, can influence both the energy consumption and the purity of the extracted metal.
One of the main benefits includes:
- Increased yield of pure metals: Efficient oxidation processes can lead to higher recovery rates and less waste, making operations more economical.
- Tailoring properties: Factors like surface area or porosity of anodes are custom-designed to maximize efficiency based on specific extraction needs.
Challenges still exist in metal extraction processes, particularly regarding anode degradation over time due to the harsh chemical environment. Thus, ongoing research seeks to enhance material resilience and longevity during these reactions.
Water Splitting for Hydrogen Production
Another significant application of anodes in electrolysis is in water splitting, which generates hydrogen and oxygen from water. This method is viewed as a clean alternative energy source, facilitating the transition away from fossil fuels. The electrolysis of water takes advantage of anodes to support the oxidation of water molecules, releasing oxygen gas while freeing up electrons.
Key considerations for this process include:
- Anode material choice: For efficient hydrogen production, anodes are often crafted from precious metals like iridium oxides or from more cost-effective materials like nickel or cobalt. This helps in boosting catalytic activity and overall efficiency.
- Impact on energy consumption: The efficiency of water splitting directly correlates to the design of the anode. Innovations, including the development of nanostructured or hybrid anodes, aim to reduce energy losses and operational costs.
"As the world seeks sustainable energy solutions, efficient hydrogen production via electrolysis may prove vital in securing a greener future."
In summary, the applications of anodes in electrolysis range from enhancing metal extraction techniques to driving the production of hydrogen. Both fields face unique challenges but also offer exciting opportunities for technological advancements and sustainability.
Emerging Trends in Anode Development
Emerging trends in anode development reflect crucial advancements in the field of electrolysis. As the demand for more efficient and sustainable methods in industrial processes increases, the role of innovative anode materials and designs becomes significantly important. Researchers and engineers are exploring new avenues to optimize electrolysis efficiency, improve performance, and reduce costs. These developments may lead to better energy utilization and enhanced overall productivity in various applications such as metal extraction and water splitting for hydrogen production.
Nanostructured Anodes
Nanostructured anodes represent a transformative approach to enhancing the performance of electrolysis systems. The unique properties of materials at the nanoscale often lead to improved electrical conductivity and larger surface area. These characteristics can facilitate greater rates of oxidation reactions, ultimately increasing the current efficiency of the electrolysis process.
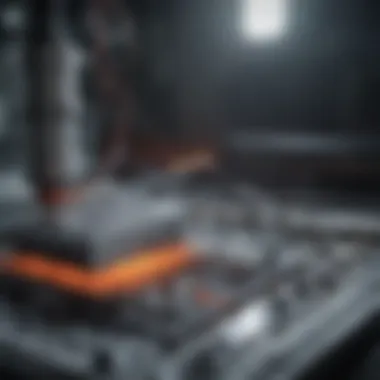
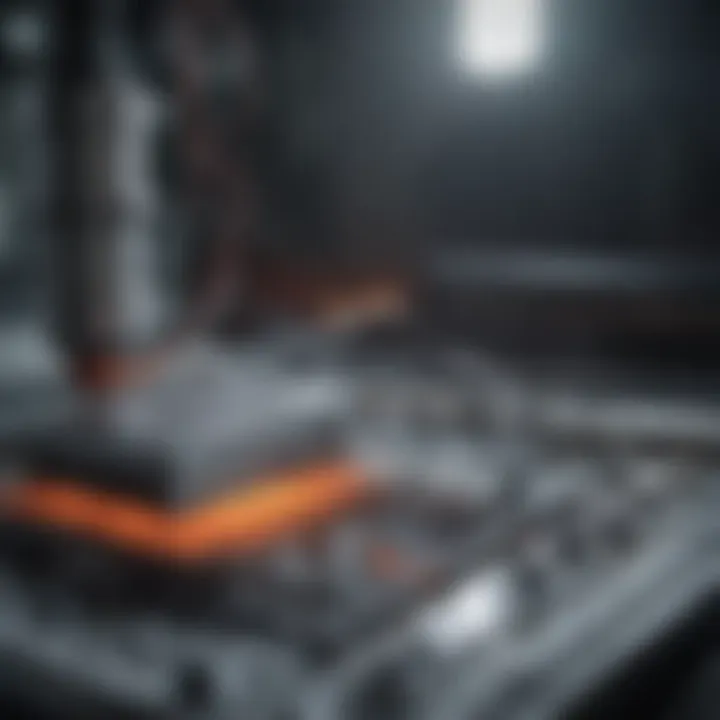
Nanostructured materials, such as carbon nanotubes and graphene, have shown promising results in laboratory settings. These materials not only enhance electron transfer rates but also exhibit resistance to corrosion and degradation. Their application can be crucial in harsh electrolytic environments, which often hinder traditional anode materials.
Furthermore, the scalability of nanostructured anodes presents both opportunities and challenges. While manufacturing at a nanoscale can be complex and costly, potential improvements in overall system efficiency may outweigh these drawbacks. Some researchers are focusing on optimizing the fabrication processes to make the production of nanostructured anodes more feasible for commercial applications.
Hybrid Anode Systems
Hybrid anode systems combine different materials or technologies to leverage the strengths of each component. This approach aims to enhance performance while minimizing the weaknesses associated with traditional anode materials. For example, systems that integrate metallic anodes with ceramic coatings can benefit from the conductivity of metals and the durability of ceramics.
These hybrid systems are designed to optimize the surface area available for chemical reactions, enhancing the efficiency of the oxidation process. As industries look for ways to optimize resource use, hybrid systems may offer a balanced solution between efficiency and material conservation.
Additionally, hybrid anode systems are being explored in the context of environmental sustainability. For instance, using recycled materials in the construction of these anodes can not only reduce costs but also minimize the ecological footprint of production. The intersection of efficiency and sustainability is becoming a priority for researchers and industries alike, guiding the development of future anode technologies.
"The future of electrolysis lies not only in efficiency but also in the sustainable use of resources in anode development."
In summary, emerging trends such as nanostructured and hybrid anode systems are crucial for the advancement of electrolysis technology. They promise improved performance, sustainability, and adaptability in various applications, ultimately shaping the future of this vital industrial process.
Challenges and Limitations
The exploration of challenges and limitations within the context of the anode in electrolysis sheds light on critical issues affecting its performance and application. Understanding these challenges is essential for researchers and professionals working in the field, as it directly impacts efficiency and scalability of electrolysis processes. The main areas of concern are the degradation of materials used for anodes and the issues arising during scale-up of electrolytic systems.
Material Degradation
Material degradation at the anode is a significant challenge that can affect the overall performance of the electrolysis process. Anodes are often subjected to harsh chemical conditions, particularly in environments with high electrolyte concentrations and elevated temperatures. Over time, this leads to the corrosion or wear of the anode material, impacting both efficiency and longevity.
Common materials like graphite or platinum suffer from oxidation, which can lead to reduced surface area and ultimately decrease the rate of oxidation reactions. Furthermore, the type of electrolyte used can also play a role in the degradation of the anode. For instance, strong acids or bases often accelerate material wear.
To combat these issues, researchers are constantly developing innovative anode materials that are more resistant to degradation. Some solutions involve using coatings or composites that enhance durability. This shift not only improves efficiency but also reduces the need for frequent replacements, contributing to more sustainable electrolysis practices.
Electrolysis Scale-up Issues
Scaling up electrolysis technologies from laboratory to industrial applications presents multiple challenges, particularly regarding the anode. Large-scale systems require uniform performance across multiple anodes. Variability in this uniformity leads to inefficiencies that are challenging to address.
Additionally, when systems are scaled up, the demands on anode materials become more pronounced. The risk of overheating, uneven current distribution, and localized degradation increases with size. Tackling these issues necessitates careful design and engineering of the anode configuration to ensure consistent performance under varying conditions.
Moreover, the economic implications of scaling up electrolysis systems cannot be overlooked. High-quality materials that resist degradation often come with a significant cost. Balancing performance and cost-effectiveness is crucial for successful implementation in commercial settings.
The successful scale-up of electrolysis processes hinges on addressing anode design, material choice, and the overall integration of these systems into existing infrastructure.
Future Directions in Anode Research
The field of electrolysis is evolving rapidly, with research focusing on innovative anode materials and methods. Future directions in anode research are critical for enhancing the efficiency, sustainability, and scalability of electrolysis applications. Understanding these directions allows researchers and industry professionals to optimize their work and effectively address the growing demand for renewable energy sources and advanced manufacturing processes.
Recent advancements in materials science have opened new pathways for developing anodes that are more efficient and durable. This exploration can lead to reduced energy consumption and lower costs in various electrochemical applications. Additionally, there is a significant focus on the environmental impact of anode materials, pushing for a shift towards greener alternatives.
Innovations in Anode Technology
Innovations in anode technology focus on creating materials that improve performance during electrolysis. One of the key elements in this innovation is the use of advanced materials such as carbon-based composites, conductive polymers, and transition metal oxides. These materials may provide enhanced electrical conductivity and better resistance to corrosion, which is vital for long-term performance.
Additionally, researchers have begun to explore the use of nanostructured materials. Nanostructures have unique properties that can significantly enhance the surface area of anodes. This increase in surface area improves the overall reaction rate, leading to higher efficiency in electrolysis processes. Research continues to validate the benefits of such materials, paving the way for their future use in commercial applications.
Moreover, the development of hybrid anode systems is gaining attention. These systems combine the characteristics of various materials to achieve increased efficiency and reduced degradation. For instance, integrating metals with conductive polymers can yield anodes that are not only effective but also sustainable in the long run.
Interdisciplinary Approaches
The pursuit of advancements in anode technology shows the importance of interdisciplinary approaches in research. Collaboration across disciplines, such as chemistry, materials science, and engineering, is crucial for fostering innovative solutions to complex problems in electrolysis. By merging knowledge and expertise, researchers can develop anodes that incorporate multifunctional capabilities, satisfying diverse industrial needs.
For example, chemists may offer insights into the molecular interactions that occur during electrolysis, while materials scientists can contribute knowledge about the mechanical properties of new materials. Engineers play a vital role in designing experiments and optimizing industrial processes to implement these advancements efficiently.
This collaborative mindset not only accelerates the development of new technologies but also enhances the sustainability of the electrolysis processes. Engaging various scientific disciplines ensures that researchers can tackle environmental impacts, enhance performance, and develop cost-effective solutions.
In summary, the future of anode research is positioned at the intersection of innovation and interdisciplinary collaboration. By leveraging advancements in technology and fostering teamwork among diverse fields, we can improve the efficacy of electrolysis and contribute to a more sustainable future.
End
The conclusion of this article is critical as it encapsulates the essential findings regarding the role of the anode in electrolysis. It serves as a final piece that ties together all discussions presented throughout the sections. Understanding how the anode operates is vital for anyone engaged in electrochemical processes. This understanding not only informs theoretical knowledge but also has profound implications for practical applications in various fields such as metal extraction and energy production.
Summary of Key Findings
The examination of the anode reveals several key insights:
- Functionality: The anode's primary role is to facilitate oxidation reactions, where electrons are removed from the substances involved. This process drives the electrolysis forward.
- Materials: Various materials can be utilized for anodes, each offering different properties that influence performance and durability. Common materials include graphite and platinum, while innovations include nanostructured and hybrid systems, which enhance efficiency.
- Electrochemical Mechanisms: A deep dive into the mechanisms clarifies how electrons move towards the anode and how these movements affect the overall efficacy of the electrolysis process.
- Challenges: Material degradation and issues related to scaling up electrolysis operations were also critical points. These challenges must be addressed to optimize the use of anodes in industrial applications.
Final Thoughts on Anodes in Electrolysis
By focusing research on optimizing anode materials and designs, it is possible to enhance the efficiency of electrolysis processes, paving the way for more sustainable practices in areas like hydrogen production and metal recovery. This research not only contributes to academic knowledge but also holds promise for significant industrial applications that can benefit society as a whole.