Exploring Advances in Multi Electrode Array Electrophysiology
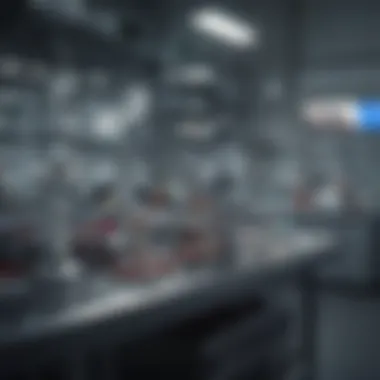
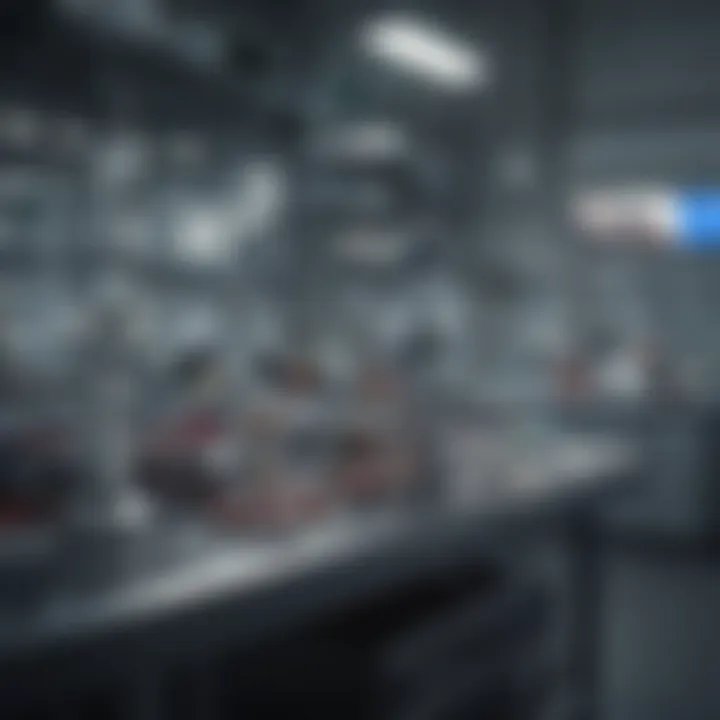
Intro
In the landscape of neuroscience research, the ability to monitor and manipulate neuronal activities is vital. Multi electrode array electrophysiology (MEA) serves as a cornerstone in this pursuit, offering a sophisticated means to explore interactions within neuronal networks. This approach has evolved significantly, with developments in technology leading to new techniques and methodologies that enhance research quality and data fidelity.
Multi electrode arrays consist of an arrangement of electrodes that can simultaneously record electrical signals from multiple sites within a sample, providing rich data that traditional methods cannot achieve. This capability opens avenues for investigating both fundamental biology and potential therapeutic applications.
Through this article, readers will gain insight into the essential principles of MEA technology, its advancements, and practical applications in both academic and clinical settings. As we dive into the world of MEAs, we aim to outline their significance and how they shape our understanding of neuronal behavior and pharmacological responses.
Prelims to Multi Electrode Array Electrophysiology
Multi Electrode Array (MEA) electrophysiology has emerged as a cornerstone technique in neuroscience and cell biology, enabling unparalleled insights into the electrical activity of neurons and other cells in real time. This introduction aims to underline the importance of MEA technology, providing a foundation for understanding its intricate applications and the breadth of research it facilitates. With the capacity to record from multiple sites simultaneously, MEAs enhance the resolution and depth of data acquisition, revealing dynamics of cellular responses that single-electrode systems cannot achieve.
The significance of multi electrode arrays lies not only in their ability to capture complex biological signals but also in their transformative impact on both basic research and practical applications. Innovative designs and materials employed in MEA manufacture allow for a variety of configurations that can be tailored to specific experimental needs. Researchers benefit from viewing cellular behavior in a more holistic manner, where interactions can be observed concurrently rather than in isolation.
Definition and Significance
Multi Electrode Arrays are devices equipped with numerous electrodes arranged in a specific pattern on a substrate. These electrodes can penetrate or sit atop cells, facilitating measurements of action potentials, local field potentials, and other electrical signals that occur in biological tissues. The significance of MEAs is pronounced in areas that require detailed mapping of electrical activity, such as in studies of neurophysiology, drug effects on heart cells, and cellular networks.
The ability to monitor a large number of signals in parallel opens avenues for robust data analysis. This becomes crucial in understanding neural coding and the effects of pharmacological treatments in various diseases. Each electrode generates data that can be analyzed to extract relevant patterns, correlations, and responses, fostering a deeper understanding of cellular communications.
Historical Development
The journey of Multi Electrode Array technology began in the late 20th century when researchers sought efficient means to gather electrical signals from neurons. Early systems involved a limited number of electrodes, often leading to cumbersome experiments and inefficient data collection. As demand surged for higher resolution and expanded capabilities, innovations in microfabrication techniques came into play.
Advancements such as silicon-based substrates and advanced electrode design have greatly increased the number of recording sites per device. Improved signal processing and data acquisition technologies have further enhanced experiment quality.
Today, MEAs come in various forms and configurations, including planar MEAs, 3D MEAs, and flexible MEAs that cater to different research needs. Their evolution reflects a broader trend towards integrating engineering design with biological research, allowing for a more nuanced exploration of cellular behavior.
The development timeline of MEAs showcases landmark milestones such as:
- The first use of planar electrode arrays in neurophysiology.
- The introduction of flexible MEAs for in vivo applications.
- Integration of MEAs with optical imaging techniques.
This historical context sets the stage for comprehending the current advancements and applications of MEA technology in ongoing research.
Fundamentals of Electrophysiology
Understanding the fundamentals of electrophysiology is crucial as it lays the groundwork for harnessing multi electrode array technology effectively. Electrophysiology pertains to the study of electrical properties of biological cells and tissues, primarily focusing on how cells communicate through electrical signals and how these signals influence cellular behavior. By mastering these basic principles, researchers can gain deeper insights into neural dynamics and uncover the mechanisms behind various physiological and pathological conditions.
Basic Principles of Electrophysiology
Electrophysiology revolves around different concepts that explain how cells, particularly neurons, transmit electrical impulses. At its core, the resting membrane potential, which describes the voltage difference across the membrane of a cell when it is not actively transmitting signals, is a fundamental concept. This potential arises due to the unequal distribution of ions, primarily sodium and potassium, inside and outside the cell.
When a neuron receives sufficient stimulus, it undergoes depolarization. This process involves a rapid influx of sodium ions, leading to a change in membrane potential that activates action potentials. The action potential then propagates along the nerve fiber until it reaches synapses, where neurotransmitters are released, facilitating communication with neighboring neurons.
Other essential concepts include the role of ion channels, which regulate ion flow and determine excitability, and synaptic transmission, the process by which signals are transmitted between neurons. These principles provide a framework for interpreting the results obtained from multi electrode array experiments.
Types of Electrophysiological Measurements
Electrophysiology encompasses various measurements that can be conducted to analyze cellular and neural activity. The most common measurement types include:
- Intracellular Recording: This measurement captures the electrical activity within a single cell by inserting a microelectrode into the cell.
- Extracellular Recording: This technique records signals from multiple cells within a certain vicinity using multi electrode arrays. It provides a broader view of neuronal communication and network dynamics.
- Whole-Cell Recording: It combines aspects of both intracellular and extracellular recordings and allows detailed studies on ion channel activity.
- Field Potential Recording: This measures the summed electrical activity of a group of neurons, providing insights into the functional dynamics of neural circuits.
The integration of these measurements is valuable when designing experiments using multi electrode arrays. They enhance the understanding of the effects of chemical, electrical, or pharmacological manipulations on neuronal behavior, paving the way for advancements in neuroscience research.
"Electrophysiological techniques have been pivotal in elucidating the complex mechanisms of neuronal communication and behavior, essential to advancements in neuroscience and related fields."
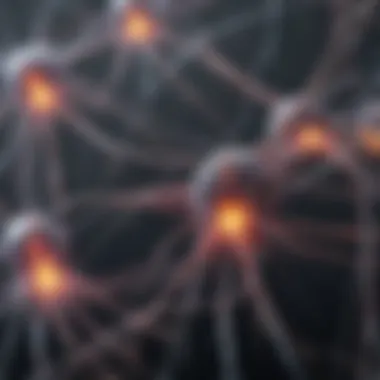
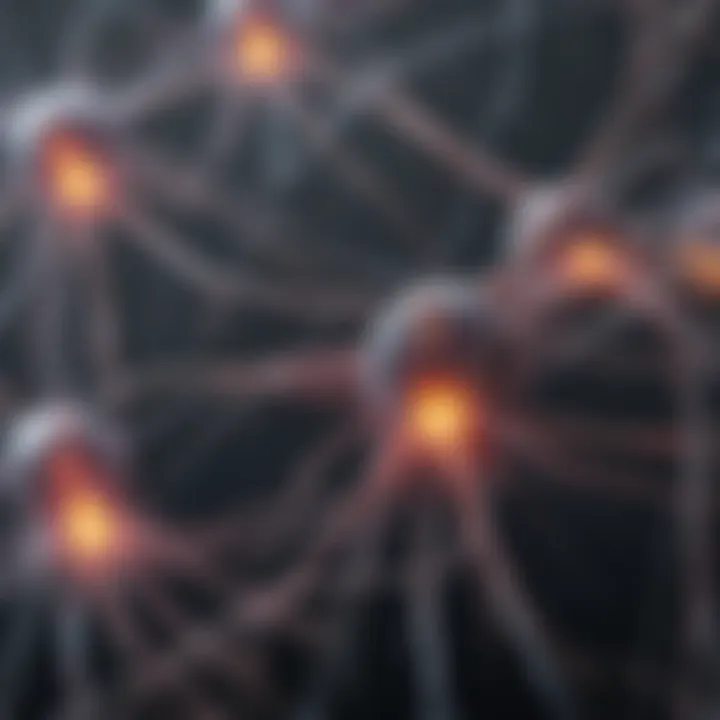
Design and Components of Multi Electrode Arrays
The design and components of multi electrode arrays (MEAs) are critical for the success of electrophysiological experiments. Understanding these aspects allows researchers to make informed choices that can greatly enhance the quality of data collected during experiments. A robust design is essential, as it dictates how parameters like spatial resolution, signal fidelity, and integration with biological tissues are achieved. Each component plays a part in determining overall system performance.
Structure of MEAs
The structure of multi electrode arrays typically consists of a substrate onto which electrodes are patterned. This layout is designed with precision and can vary depending on the application. Common substrates include silicon, glass, or flexible polymers. The geometry, density, and arrangement of electrodes are crucial for their effectiveness. For example, a higher density of electrodes can increase the spatial resolution of recordings, while a specific arrangement can enhance interaction with biological samples.
An important design consideration is the electrode size, which often ranges from tens to hundreds of micrometers. Smaller electrodes may detect signals with higher resolution, but they can also be more sensitive to noise. Thus, a balance must be struck in design choices.
Types of Electrodes Used
Electrodes used in MEAs can be classified into several types, each with unique characteristics.
- Metal electrodes: These are commonly used due to their reliability and good conductivity. Materials like platinum and gold are common choices. They are robust and provide stable signals but may have limitations in long-term cell interfacing.
- Carbon-based electrodes: Growing in popularity, these electrodes offer benefits such as biocompatibility and reduced noise levels. They can be made from various forms of carbon, including carbon nanotubes or graphene, which provide enhanced electrical properties.
- Conducting polymer electrodes: These materials have the advantage of increasing biocompatibility. Conducting polymers can align with biological environments better than metals, making them suitable for long-term studies involving living cells.
Choosing the right type of electrode can depend heavily on the specific application and desired outcomes.
Materials and Fabrication Techniques
The materials and fabrication techniques utilized in the production of MEAs are vital for their overall performance and functionality. The choice of materials affects not only the mechanical properties but also the electrical characteristics of the electrodes. Common materials include silicon, which is often used due to its properties as a semiconductor, and various polymers that can offer flexibility and biocompatibility.
Fabrication techniques have evolved significantly. Traditional methods like photolithography have largely been used, but newer techniques incorporate soft lithography and 3D printing, which allow for more complex designs. 3D printing, in particular, provides the advantage of creating custom shapes and orientations of electrodes, enabling tailored solutions for specific applications.
Advances in fabrication techniques enhance the ability to create high-resolution MEAs, which is essential for exploring phenomena like synaptic plasticity and cellular dynamics.
Selecting appropriate materials and techniques ensures that the MEA can withstand environmental conditions and maintain high electrical performance. This selection process is fundamental to maximizing data quality and achieving accurate interpretations in electrophysiological studies.
Data Acquisition and Analysis
Data acquisition and analysis are critical components in the realm of multi electrode array electrophysiology. These processes facilitate the collection, processing, and interpretation of neural signals recorded from the arrays. Understanding how to effectively gather and analyze data directly influences the outcomes of experimental research and the subsequent insights derived from it. Accurate data acquisition ensures that the information obtained truly reflects the biological activity being studied while appropriate analysis techniques harness the full potential of that data. In this section, we will explore the significance of data acquisition and analysis, discuss signal processing techniques, and delve into statistical analysis methods employed in electrophysiology.
Signal Processing Techniques
Signal processing techniques are essential in the manipulation of raw data recorded from electrode arrays. The aim of these techniques is to extract meaningful patterns from noise that often accompanies biological signals. Common methods include filtering, amplification, and digitization of signals. Each technique serves a unique purpose in data refinement.
- Filtering: By applying low-pass, high-pass, or band-pass filters, researchers can eliminate unwanted frequencies. This step is crucial as it helps to remove electrical noise and other artifacts that could skew the results.
- Amplification: Bioelectric signals are typically of low amplitude; therefore, amplifying them is necessary for accurate detection. Operational amplifiers and other amplification systems are commonly used to enhance signal strength for improved interpretation.
- Digitization: Once the signals are filtered and amplified, they need to be converted into digital format for further analysis. This is done through an analog-to-digital converter (ADC), allowing for sophisticated processing using algorithms.
These processing techniques not only enhance signal quality but also aid in the interpretation of the data collected, ensuring that the findings are robust and reliable.
Statistical Analysis in Electrophysiology
Statistical analysis in electrophysiology is pivotal for drawing conclusions from data collected through multi electrode arrays. The quantitative nature of the data demands rigorous assessment to ensure validity and reproducibility of research findings. Key statistical techniques include:
- Descriptive Statistics: These summarize the basic features of the data, providing simple summaries such as means, medians, and standard deviations. Descriptive statistics are essential for understanding the general trends present in the data.
- Inferential Statistics: To draw conclusions that extend beyond the immediate data, inferential statistics come into play. Tests such as t-tests, ANOVA, and regression analysis allow researchers to make predictions or infer correlations from data.
- Data Visualization: Representing data graphically can enhance understanding. Techniques such as histograms, scatter plots, and heat maps effectively convey complex data insights, highlighting patterns or outliers that warrant further investigation.
- Modeling: Advanced modeling techniques, including Bayesian models or machine learning algorithms, are increasingly applied in electrophysiological research. These help predict outcomes based on existing data and can uncover relationships that traditional methods might overlook.
"Accurate data acquisition and thorough analysis are cornerstones of reliable findings in electrophysiology."
Applications of Multi Electrode Arrays
Multi electrode arrays (MEAs) represent a pivotal technology in electrophysiology, offering diverse applications across various scientific disciplines. These applications demonstrate the significance of MEAs in enhancing our understanding of complex biological systems. The capacity to record and stimulate multiple sites simultaneously provides unparalleled insights into neuronal networks and cellular behaviors. This section elucidates the applications of MEAs, emphasizing their relevance and implications in research and industry.
Neuroscience Research
In neuroscience, multi electrode arrays serve as crucial tools for investigating brain activity and functionality. They enable researchers to record electrophysiological signals from multiple neurons concurrently. This parallel recording capability is particularly valuable for studying neural circuits and understanding how they contribute to various cognitive functions.
MEAs facilitate the exploration of phenomena such as synaptic plasticity, network oscillations, and neural dynamics. For example, researchers have employed these arrays to study the spatiotemporal patterns of electrical activity during learning processes. Moreover, MEAs allow for the observation of how different neuron types interact in real time, providing insights into the complexities of neural communication.
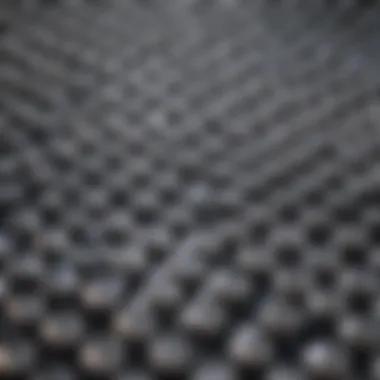
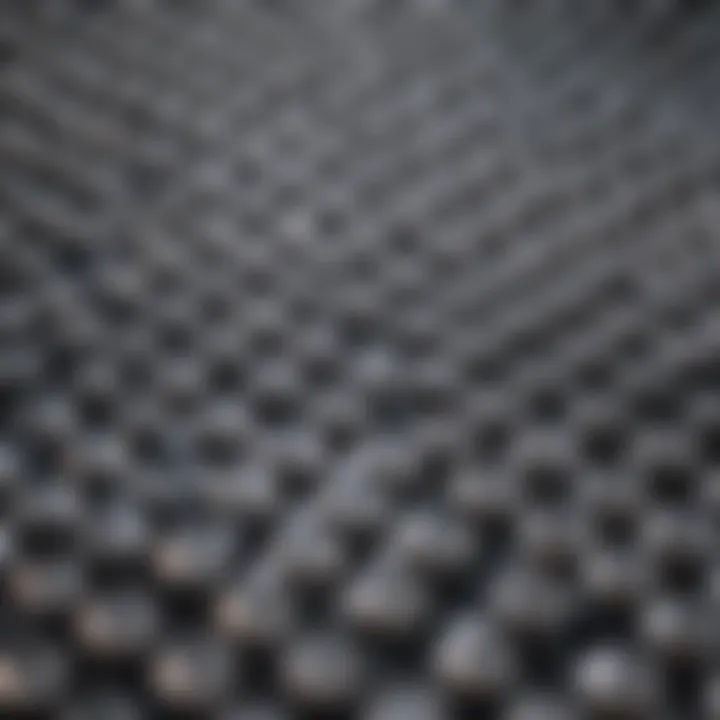
The implementation of MEAs in live brain tissue and organotypic slices allows researchers to observe biological activities in a more natural environment. This capacity can lead to breakthroughs in understanding neurological disorders and the development of targeted therapies.
Drug Discovery and Pharmacology
In the field of pharmacology, MEAs play a pivotal role in drug discovery processes. These arrays allow for high-throughput screening of compounds by measuring the effects of drugs on neuronal activity. This method facilitates rapid assessment of drug efficacy and toxicity. The ability to simultaneously evaluate numerous drugs on various cell types accelerates the discovery pipeline, thus becoming essential in early-stage drug development.
Using MEAs, researchers can also study the mechanisms of action of pharmacological agents at a cellular level. By monitoring changes in neuronal firing rate or patterns, scientists can infer how a drug affects specific neural circuits, which is crucial information for drug targeting. Furthermore, the integration of MEAs with automated platforms enhances the throughput of pharmacological studies, making it easier to identify promising candidates for clinical trials.
Tissue Engineering
Tissue engineering is another area where multi electrode arrays demonstrate immense potential. MEAs can be combined with engineered tissues to create living systems that mimic natural physiological conditions. This integration allows for studying cellular responses in engineered tissues, making MEAs invaluable in developing functional tissue substitutes.
By measuring electrical signals from engineered tissues, researchers can evaluate the health and functionality of these constructs. This application is particularly significant for cardiac and neuronal tissues, where electrical activity is a critical indicator of tissue performance. Moreover, MEAs enable the assessment of how changes in the microenvironment influence cell behavior and function. This capability can inform strategies for improving tissue viability and integration into host systems.
The integration of MEAs in tissue engineering not only advances research but may eventually lead to clinical applications in regenerative medicine.
The applicability of multi electrode arrays extends beyond these areas, reaching into domains like neuroprosthetics and disease modeling. As MEA technology continues to evolve, its role in both basic and applied research is likely to expand, opening new avenues for scientific inquiry and innovation.
Advancements in Multi Electrode Array Technology
Advancements in multi electrode array technology play a crucial role in enhancing our understanding of cellular behavior and neural activities. These innovations contribute significantly to both the accuracy of measurements and the breadth of applications in scientific research. By merging novel materials and refined manufacturing processes, researchers can increase the sensitivity and resolution of multi electrode arrays (MEAs). This development empowers scientists to capture subtle variations in electrical activity across neural circuits. Moreover, as MEAs become more versatile, they open doors to interdisciplinary approaches and novel experimental designs.
Emerging Technologies in MEAs
Emerging technologies are reshaping the landscape of MEA applications. For instance, recent advancements in nanotechnology have introduced nanoscale electrodes that provide higher spatial resolution. This enhancement allows for recording from smaller networks of neurons without compromising the integrity of the biological samples. Additionally, wireless communication systems now enable real-time data transfer. These systems minimize disturbances to the sample while allowing for continuous monitoring.
Another promising approach involves using flexible and biocompatible materials. These materials adapt better to biological environments, reducing the likelihood of immune responses that can negatively impact recordings.
- Key points of emerging technologies include:
- Nanoscale electrodes for improved resolution.
- Wireless data transmission for non-invasive monitoring.
- Flexible materials that align with biological systems.
Integration with Other Techniques
The integration of MEAs with other investigative techniques enhances the depth and context of data collected. For example, combining MEA technology with calcium imaging offers a more comprehensive view of neuronal signaling. Calcium imaging tracks intracellular calcium levels, which are critical for understanding neuronal excitability. The simultaneous use of these techniques allows for a detailed correlation between electrical and chemical activities in neural networks.
Another notable integration involves coupling MEAs with optogenetics. This method enables precise control over neuronal activity with light. By integrating these technologies, researchers can manipulate and monitor the same population of neurons, providing insights into causal relationships and functionalities within neural circuits.
- Important considerations in integration:
- Enhances data richness and context.
- Facilitates understanding of complex neuronal function.
- Promotes interdisciplinary research pathways.
"Integrating multi electrode arrays with modern techniques may unveil unprecedented insights into neurobiology, improving our grasp of both normal and pathological conditions."
Challenges and Limitations
Understanding the challenges and limitations inherent in multi electrode array electrophysiology is crucial for researchers aiming to refine this technology and its applications. While the advancements in MEA technology have paved the way for groundbreaking discoveries in neuroscience and pharmacology, several hurdles still persist. Addressing these issues not only enhances the reliability of data collected but also improves the design and implementation of experiments.
Technical Challenges in MEA Implementation
Implementing multi electrode arrays involves several technical difficulties that can compromise the quality of the data generated. One fundamental challenge is the resolution of the recording electrodes. Many existing MEAs struggle to achieve the spatial resolution necessary to capture the nuanced activity of small neuronal populations. Poor resolution can lead to a significant loss of critical information, making it difficult to interpret the results accurately.
Moreover, signal-to-noise ratio (SNR) is another prominent issue. Environmental factors, as well as intrinsic system noise, can interfere with the electrical signals picked up by the electrodes. This limitation necessitates advanced signal processing techniques to filter out unwanted noise before analysis can begin, complicating the setup further.
Powering the MEAs also presents challenges. Continuous operation can lead to thermal drift, affecting the sensitivity and stability of the recordings. Engineers must work diligently to develop designs that mitigate heat generation while ensuring integrity of signal acquisition.
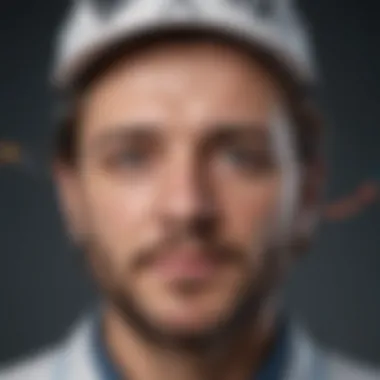
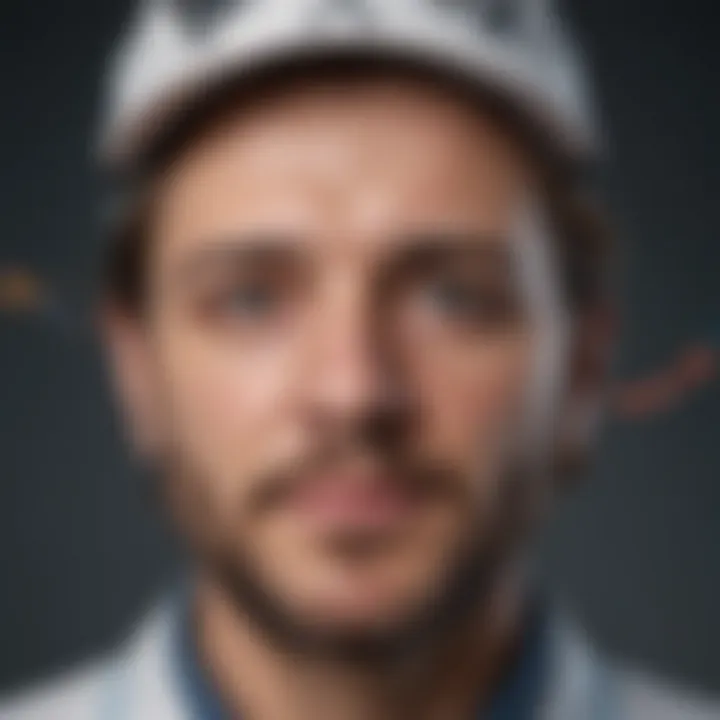
Inadequate scalability of current MEA systems also poses limitations to widespread application. Researchers often require MEAs that can interface with larger cell populations but struggle to find systems that can seamlessly scale without losing functionality. These factors collectively underscore the complexities of successful MEA implementation.
Biological Variability and Data Interpretation
Biological variability is an inevitable aspect of electrophysiology and comes with its own set of challenges. Different cells and tissues possess unique electrical properties, inherently introducing variability into the results. This diversity can lead to disparities in data that make interpretation difficult. Researchers must establish robust controls to ensure that differences observed are truly due to experimental variables rather than biological noise.
Additionally, data interpretation can be clouded by the multifactorial nature of biological systems. Responses to external stimuli can differ significantly among individual cells, complicating the development of generalized conclusions. Researchers must rely on extensive statistical models to extract meaningful insights from their data. This process is time-consuming and can introduce its own biases into the interpretation.
Understanding these challenges emphasizes the necessity for continued innovation and methodical exploration in multi electrode array research. Finding ways to enhance the technology and address its limitations will ultimately foster better experimental outcomes.
Future Directions in Multi Electrode Array Research
The future of multi electrode arrays (MEAs) in electrophysiology is a vital area of exploration. It embodies the interplay between innovation and application, offering significant opportunities to understand complex biological systems. A focus on future directions helps researchers and professionals gauge where advancements will likely lead and how these changes can enhance research productivity and accuracy. As the field evolves, new methodologies combined with existing frameworks promise to create a more profound understanding of neuronal and cellular behavior.
Innovative Research Approaches
There is a noticeable shift toward innovative research approaches in the use of MEAs. Integrating alternative signal processing techniques presents a powerful way to enhance data resolution. Use of machine learning algorithms can improve the classification of neural signals. This can lead to better insights into how neural networks operate, what patterns signify neural diseases, and how drugs affect cellular behavior.
Moreover, developing next-generation MEAs with enhanced spatial and temporal resolution is essential. Innovations in microfabrication techniques, such as 3D printing, create custom electrode arrangements, allowing researchers deeper insights into precise cellular environments. The application of hierarchical electrode structures, where multiple layers interact with various depths of tissue, presents a way to study dynamic cellular processes more effectively.
Research in bioengineering is also advancing MEAs significantly. For example, the incorporation of biocompatible materials may lead to longer term and more stable interactions with living tissues. Exploration in this domain expands the potential of MEAs in chronic recordings, which is crucial in the study of neurodegenerative diseases.
Potential Interdisciplinary Collaborations
The future direction of multi electrode arrays may be considerably enriched through interdisciplinary collaborations. Ties with fields like computer science, nanotechnology, and materials science could lead to breakthroughs unachievable in isolation. For instance, computer science can enhance data analytics capabilities, permitting more sophisticated interpretations of complex datasets.
Moreover, collaboration with materials scientists can yield novel electrode materials enhancing conductivity, biocompatibility, and stability. This can directly support the lifetime of MEAs in practical applications.
Additionally, partnerships with pharmacologists are essential. This will enable the integration of pharmacological studies with electrophysiological measurements. Understanding how drugs interact with neuronal circuits will improve drug discovery and development processes, facilitating the rapid translation of discoveries from bench to bedside.
By uniting expertise from various disciplines, the next generation of multi electrode arrays could revolutionize our understanding of neuroscience and significantly impact therapeutic interventions.
Culmination
The conclusion of this article encapsulates the core elements of Multi Electrode Array (MEA) electrophysiology. This technique is not just a technological marvel; it represents a significant leap in our ability to probe neural dynamics and cellular behaviors. Among its numerous benefits, MEA technology offers high-resolution data collection, enabling researchers to observe real-time physiological activity across multiple sites. This multifaceted approach enhances the understanding of complex biological systems, thereby contributing to advancements in neuroscience, pharmacology, and tissue engineering.
Considerations about the future of MEA research are equally important. As we conclude, it is vital to recognize that ongoing advancements in materials and sensor technology will likely enhance the sensitivity and applicability of MEAs. Moreover, the integration of AI and machine learning into data analysis stands to revolutionize how researchers interpret vast datasets, uncovering patterns and making predictions with much greater accuracy.
"The prospective benefits of MEA technology extend beyond basic research; they are poised to impact clinical applications and therapeutic strategies significantly."
Summary of Key Insights
In reviewing key insights from the discussions throughout this article, several critical points emerge:
- Technological Development: Advancements in fabrication materials and design have made MEAs more flexible and applicable to a variety of cell types and experimental conditions.
- Applications in Research: MEAs have shown profound utility in both basic research and applied sciences, supporting studies in areas such as neurodevelopment and drug responses.
- Challenges Faced: While MEAs provide rich data, researchers must navigate challenges related to biological variability and data interpretation complexities.
Implications for Future Research
The future of Multi Electrode Array technology presents exciting opportunities for interdisciplinary collaborations. Potential research directions include:
- Integration of MEAs with in vivo studies: This could enhance understanding of brain functions.
- Emerging Techniques: The pairing of MEAs with optogenetics may lead to breakthroughs in manipulating neuronal activity.
- Enhanced Data Analytics: Employing sophisticated algorithms and machine learning to analyze MEA data offers a pathway for more profound insights into cerebral networks.
As we harness these strategies, it is clear that MEAs will remain at the forefront of electrophysiological research, unlocking further understanding of complex biological processes and contributing to the development of novel therapeutic interventions.
Importance of References in This Article
- Credibility: Using references enhances the credibility of the article. By citing original research, theories, and established authors in the field, the work becomes more authoritative. The reader knows that the information is backed by preceding studies and can trust the conclusions drawn from them.
- Contextualization: References help situate multi electrode arrays within the broader field of electrophysiology. They provide context for how this technology has evolved, its applications, and its limitations. An understanding of previous work informs current research directions and sets the stage for future innovations.
- Facilitating Further Research: Including references allows readers to delve deeper into specific topics. Researchers, students, and educators can trace back to the primary sources and engage with the original findings. It encourages the exploration of themes that may be only briefly touched upon within the article.
- Attribution of Ideas: A good practice in academia is to attribute ideas and findings to their original authors correctly. This not only shows respect for intellectual property but also aids in the academic discourse, allowing for the exchange of knowledge.
- Showing the Evolution of the Field: References can illustrate how multi electrode arrays have been utilized over time, revealing shifts in methodologies, technologies, and areas of application. Understanding these changes is crucial for grasping where the field stands today and where it may be headed.
Considerations about References
- Staying Current: It's important to include recent sources to reflect the latest advancements in multi electrode array technology. Technologies evolve rapidly, and thus, relying solely on older publications may present an outdated view of the field.
- Diversity of Sources: Citing a variety of references, including journal articles, conference papers, and authoritative books, creates a balanced and comprehensive narrative. Different sources can offer unique perspectives and insights.
"The study of multi electrode array technology has transformed our understanding of neural networks and cellular interactions. By continuously engaging with new literature, researchers can stay at the forefront of this evolving field."